Familial hypercholesterolemia (FH) is categorized among autosomal dominant disorders [1], which in time can trigger cardiovascular disease [2–4]. In this abnormality, most cases are caused by a loss-of-function mutation in the gene coding for the low-density lipoprotein receptor (LDLR) [5, 6]. The LDLR plays a critical function in the elimination of cholesterol from the circulation. The binding of low-density lipoprotein cholesterol (LDL-C) to LDLR initiates receptor-mediated endocytosis [2]. Furthermore, mutations in the LDL receptor-binding region of apolipoprotein B (APOB) and the infrequent gain-of-function of the proprotein convertase subtilisin/kexin type 9 (PCSK9) gene may be responsible, because binding of this protein to LDLR causes it to be degraded in lysosomes, reducing LDLR’s longevity [7]. However, this mutation is less frequent [8]. In addition, a mutation in signal-transducing adaptor family member 1 (STAP1) was also reported to cause FH [9].
The majority of FH people are heterozygous for mutations, with a prevalence of 1 in 200 [10]. In rare cases, people with FH have a homozygous genotype (both alleles have the same mutation), with a frequency of 1 in 1 million [11, 12].
Statins are the most commonly prescribed medications for the treatment of FH [13]. Statins can delay or even reverse the progression of coronary and cerebral atherosclerosis since they can increase the level of hepatic LDLR. Furthermore, statins possess numerous salutary effects that are independent of lipid lowering [14–22]. Nevertheless, some patients are statin-resistant because they do not achieve the required LDL-C goal level and continue to have cardiovascular events [23], while others are statin-intolerant and have side effects. As a result, more effective therapies to lower blood LDL-C levels to avoid atherosclerosis and its consequences are desperately needed [13].
miRNAs are regulatory molecules made up of noncoding nucleotides [24] that control gene expression by targeting complementary sequences found mostly in messenger RNAs’ 3′-untranslated region (3′-UTR), resulting in mRNA destruction or translational suppression of targeted transcripts [25]. miRNAs have been found to have therapeutic potential in the treatment of a variety of disorders, including tumors, infections, and chronic cardiovascular conditions [5, 26, 27].
Several studies have shown that miRNAs are key components of the cardiogenic regulatory network [28, 29]. The discovery of putative target genes for these FH-associated miRNAs could assist us in a better understanding of FH progression. miRNA-27a and miRNA-27b are two isoforms of the miRNA-27 family. miRNA-27a is an intergenic miRNA, while miRNA-27b is an intronic miRNA found within the 14th intron of the human C9orf3 host gene, and miRNA-27b is also found in a mouse orthologue of C9orf3. miRNA-27a and miRNA-27b are homologous to each other, sharing 20 of the 21 nucleotides. Recently, evidence of miRNA-27’s function in the pathophysiology of cardiovascular disease has emerged. It was demonstrated that the miRNA-27 family directly targets the 3′-UTR region of ATP-binding cassette transporter A1 (ABCA1). ABCA1 is a critical mediator of apoA1 for HDL formation. It has been shown that ABCA1 is important in avoiding the accumulation of cholesterol in macrophages, while deficiency or mutation in this mediator promotes cholesterol efflux problems and leads to atherosclerosis [30]. The ability of ABCA1 to facilitate the efflux of cholesterol from peripheral cells, especially cholesterol-laden macrophages in atherosclerotic plaques, is a critical anti-atherosclerotic mechanism [31]. Regarding the study mentioned above, miRNA-27a/b can modulate apoA1-mediated cholesterol efflux by decreasing the expression of ABCA1. Furthermore, miRNA-27a has been reported to target the PCSK9 gene’s promoter region and upregulate its expression. Considering these findings, the present study aimed to investigate the association of miRNA-27a/b with FH patients and the severity of the disease based on the zygosity status.
Material and methods
Characteristics of study groups
The study group consisted of 39 patients (20 people with heterozygous FH and 19 with HoFH from all over Iran). A control group of 20 healthy people was also included. All participants signed a written consent form. The Mashhad University of Medical Sciences Ethics Committee accepted the study protocol. For patients with symptoms of FH, including those with high cholesterol and a family history of premature cardiac events, the Dutch Lipid Clinic Network Criteria were used to generate FH scores. The diagnosis was then completed with DNA testing to identify mutations in the LDLR, ApoB, and PCSK9 genes by next-generation sequencing methodology and verified by the Sanger sequencing method. Some of the mutations had been identified and listed as pathogenic in the ClinVar database, but others were unique mutations whose pathogenicity was predicted using the SIFT database and PolyPhen software. All patients’ information, including demographic data including age, gender, and medical history, was recorded, and blood samples were isolated. Demographic data are shown in Table I. Blood-serum samples were drawn from all patients and then allowed to clot before centrifugation. The sera were extracted, aliquoted, and stored at 80°C until they were tested.
Table I
Baseline characteristics of subjects
[i] Data are shown as mean ± SE, a significant in comparison with HoFH group, bsignificant in comparison with HeFH group. HDL-C – high-density lipoprotein cholesterol, HeFH – heterozygous familial hypercholesterolemia, HoFH – homozygous familial hypercholesterolemia, LDL-C – low-density lipoprotein cholesterol, mmol/l – millimoles per liter, TC – total cholesterol, TG – triglyceride (*p < 0.05).
Assessment of miRNA-27 expression
RNA extraction and reverse transcription (RT)
Total RNA, including miRNA, was extracted and purified from serum samples using Bonyakhte Company reagents (BON RNA Lysis buffer, BN-0011.33, Iran) according to the manufacturer’s protocol. The quality and quantity of total RNA were measured by a NanoDrop 2000 spectrophotometer (Thermo, USA). Complementary DNA (cDNA) was synthesized from ~1 µg of total RNA for each RT reaction using the BON-miR miRNA First Strand cDNA Synthesis Kit (Bonyakhteh, Iran) following the manufacturer’s instructions. For the cDNA synthesis of the desired miRNA, a specific Stem-loop RT primer designed by Bonyakhteh was used. The cDNA was synthesized using a thermocycler device for 10 min at 25°C, 60 min at 42°C, and 10 minu at 70°C.
Quantitative real-time PCR analysis
The qPCR analysis was performed for the detection of miRNA-27a/b expression by the SYBR Green method (QPCR master mix (Bonyakhteh, Iran)). After initial denaturation for 2 min at 95°C, qRT-PCR was followed by 45 cycles at 95°C for 5 s and at 60°C for 30 s. Relative quantification was based on the cycle threshold (Ct) values generated by LightCycler (Roche). The U6 small nuclear RNA (snRNA) was used as the internal control. The relative expression levels of the miRNAs of interest were calculated by the 2−ΔΔCt method. Results were represented as fold changes (FC) in the expression of miRNAs in homo and hetero serums relative to the healthy group.
Statistical analysis
For statistical analysis, SPSS software, version 26 (IBM Corp.), was utilized. Statistical significance was defined as a p-value of less than 0.05. Differences in variables were assessed using one-way analysis of variance (ANOVA) tests among three groups (healthy, HoFH, and HeFH) or a t-test for independent samples between two groups for normally distributed variables (HoFH and HeFH) and a nonparametric Mann-Whitney test was used for abnormally distributed results. Differences in variables across groups were examined using a χ2 analysis or Fisher’s exact test. Categorical variables are provided as percentages. Pearson’s correlation analyses were carried out between different indicators and miRNA-27a/b levels. In addition, Spearman’s coefficient was used for nonparametric variables. A linear regression model was used to establish the relationship between the expression of miRNA-27a/b and age and zygosity; p-values < 0.05 were regarded as statistically significant. The Biochemical GraphPad Prism software version 9 (GraphPad Software Inc., CA, USA) was used for the statistical analysis of miRNA-27a/b expression. Two-tailed p-values of less than 0.05 were considered to indicate statistical significance.
Results
Baseline characteristics of subjects
Of the total of 59 Iranian participants, 20, 19, and 20 were categorized into HeFH, HoFH, and healthy groups, respectively (Table I). The mean age of the subjects in the HeFH, HoFH, and control groups was 33.15 ±2.09, 12.89 ±2.36, and 41.50 ±1.67 years, respectively, which were significant compared to HoFH patients. Individuals included females and males, as presented in Table I. However, there was no significant difference between the groups regarding gender. The studied groups were significantly different in terms of TC and LDL-C, and they were significantly higher in HoFH subjects in comparison with the other groups. However, serum TG level was significantly higher in HoFH compared with the control group but not different from the HeFH group. Furthermore, HDL-C levels were not different among the groups (Table I). All patients in the HoFH group exhibited xanthoma symptoms, and 21% of this group used only statins, while 68.5% took both statins and ezetimibe. However, only 30% of the HeFH group took only statins, and 70% of this group did not use any drugs (Table II).
Table II
Clinical characteristics of HoFH and HeFH groups. ***p < 0.001. Median (IQR) shown for FH score
Variables | Familial hypercholesterolemia | P-value | |
---|---|---|---|
HoFH (n = 19) | HeFH (n = 20) | ||
FH score | 25.00 (23.00–26.00) | 15.00 (15.00–15.00) | < 0.001* |
Number of patients with xanthomas symptoms | 100% | 0% | 0.000* |
Mutation %: | |||
Previously reported | 75.0% | 73.3% | 0.916 |
Novel | 25.0% | 26.7% | |
Mutation type %: | |||
Missense | 55.6% | 50.0% | 0.819 |
Truncated | 22.2% | 25.0% | |
Single nucleotide polymorphism | 5.6% | 10.0% | |
Single nucleotide variant | 11.1% | 15.0% | |
Missense, truncated | 5.6% | 0% | |
LDLR position %: | |||
Exon | 87.5% | 72.7% | 0.370 |
Intron | 12.5% | 27.3% | |
Drugs consumption %: | |||
No drug | 10.5% | 70.0% | < 0.001* |
Only statin | 21% | 30.0% | |
Statin + ezetimibe | 68.5% | 0.0% |
miRNA-27 expression
The expression of miRNA-27a/b in FH patients was significantly higher in comparison with the healthy group (fold change: 2.21 ±0.69, p = 0.001). This higher significant expression was also seen when the subgroup of HoFH patients was compared with healthy subjects (fold change: 3 ±1.19, p = 0.001). However, in comparing HeFH patients and healthy individuals, the difference in expression level was of borderline significance (fold change: 1.62 ±0.46, p = 0.059). We also evaluated the difference between the HoFH and HeFH groups, and the results showed a higher expression level in the former group (fold change: 1.84 ±1.19, p = 0.009). Moreover, in subanalyses of the results, miRNA expression showed a significant difference between males in the patient group (p = 0.001) and the FH males compared to healthy males. However, the comparison between the female populations did not show significant results (Figure 1).
Figure 1
ANOVA comparison between x-fold change expression of miRNA-27a/b in the FH subgroup and control group. Control was regarded as 1. Data are expressed as mean ± SE. A – the comparison is made in terms of the total population of heterozygous familial hypercholesterolemia (HeFH) and homozygous familial hypercholesterolemia (HOFH) with the healthy group. There is a comparison between healthy and HoFH patients, a comparison of the healthy and HeFH groups, and HoFH and HeFH together. B – This graph presents the results in terms of male gender. C – This graph depicts the data in terms of females. The figures are drawn by GraphPad Prism (version 9.0.0) software
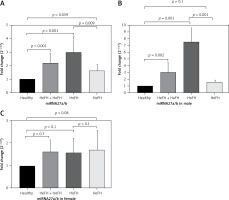
In addition, correlation analysis was also used to evaluate the association between miRNA-27 expression levels and other parameters such as age, TC, TG, LDL-C, and HDL-C, but the results were nonsignificant (Table III). In the regression analysis, FH zygosity was a significant determinant of miRNA-27 levels in the crude regression model (p = 0.039), while it had a borderline significant effect on miRNA-27 levels in the age-adjusted model (p = 0.054).
Table III
Correlation analysis between miRNA-27a/b ΔΔCt and age, FH score and lipid profile
Discussion
MicroRNAs are small RNA sequences that regulate gene expression at the post-transcriptional level by inhibiting translation or promoting the degradation of target mRNAs. These molecules have recently emerged as potential novel biomarkers for numerous diseases [15]. Circulating miRNAs play a role in controlling aging-related signaling pathways and could be exploited as new diagnostic markers for acute and chronic disorders, including cardiovascular disease [16].
In vitro experiments on mouse and human hepatoma cells have led to the discovery of a wide variety of miRNAs that directly affect LDLR expression by targeting the 3′-UTR of LDLR [15]. miRNA-27a is one of the most putative miRNAs involved in FH, as it can regulate three of the four critical photogenic genes in FH patient populations, i.e., LDLR, PCSK9, and LDLRAP1 [10].
The purpose of the current study was to investigate whether circulating levels of miRNA-27a/b are altered in FH and associated with disease severity. To achieve this purpose, we assessed the expression level of miRNA-27a/b in Iranian patients with FH, and found that serum miRNA-27a/b expression rises as a function of disease severity, in a way that HoFH patients had significantly higher levels of miRNA-27a/b expression than HeFH participants and the healthy group. This difference was also seen between the HeFH group and the healthy people. These results confirm prior findings that revealed an association of miRNA-27 with reduced LDLR protein levels and PCSK9 upregulation.
Inhibition of miRNA-27a/b has been reported as an efficient strategy for upregulation of LDLRAP1 protein and enhancing LDLR activity, leading to improved release of LDL-C from the serum of hypercholesterolemic patients [32]. In addition, previous studies have shown that miRNA-27a reduces LDLR protein levels indirectly via upregulating PCSK9, an enzyme that promotes hepatic LDLR breakdown [13]. Overexpression of miRNA-27a resulted in a rise in PCSK9 mRNA and secreted PCSK9 protein. In contrast, inhibition of miRNA-27a with LNA (locked nucleic acid) resulted in a dose-response reduction in PCSK9 levels by up to 50% compared to the control.
In HoFH patients, additional LDL-C reduction can be achieved by inhibiting the production of LDL-C or its precursors with either lomitapide, a microsomal triglyceride transfer protein (MTP) inhibitor, or mipomersen, an antisense oligonucleotide that inhibits apoB synthesis [33].
Regular LDL-C apheresis, which manually removes LDL-C, can also assist in decreasing LDL-C levels. The most extensively used cholesterol-lowering medications, statins, and ezetimibe, are now generically available in most countries at a reasonable cost [34]. Despite the 50–60% LDL-C decrease achieved by these two typically well-tolerated medications, a considerable number of FH patients fail to meet the prescribed LDL-C objectives due to their extremely high baseline LDL-C levels [35]. On traditional medication therapy, less than 10% to 20% of HeFH patients with signs of CVD reach an LDL-C level below 70 mg/dl [36]. Even high doses of atorvastatin reduce mean LDL-C by only 22–25% in HoFH patients, with untreated LDL-C > 500 mg/dl and only minimal to moderate residual LDLR activity, and ezetimibe achieves an additional 20% reduction [37], so few, if any, HoFH patients can achieve anywhere near optimal LDL-C levels. As a result, alternative therapeutic targets and medicines are being evaluated to lower the residual disease burden in individuals with FH [38–41], either alone or in combination with currently approved therapies. Regulation of miRNA-dependent gene expression appears to be a potential approach in this context.
In view of the involvement of miRNA-27a/b in regulating various pathways in FH, inhibiting miRNA-27a may be regarded as a potential therapeutic option. In addition, we assessed the correlation between age and gender to start the FH process. The participants with homozygous FH had an average age of 12.8 ±2.36 years, which was lower than the HeFH and healthy groups. As a result of their severe cardiovascular problems, individuals with homozygous features do not live long lives and face early morbidity and mortality.
Some limitations are worthy of attention for the current study. First, the population size was relatively small owing to the fact that the diagnosis of FH was based on genetic confirmation. Therefore, larger scale analyses are warranted to allow diagnostic accuracy testing. Moreover, the case-control design of the current study did not allow us to examine the possible association between circulating miRNA-27a/b levels and cardiovascular complications of patients in a longitudinal manner. Finally, the small population studied precluded the possibility of a detailed assessment of the possible impact of lipid-lowering therapies on circulating miRNA-27a/b levels, and whether the levels of this miRNA can assist monitoring the efficacy of treatment.
In conclusion, we observed a higher level of miRNA-27a/b in patients with FH compared to healthy individuals and in the HoFH group compared with the HeFH group. In addition, by examining the results of comparing miRNA-27a/b in both sexes, it can be concluded that this disease is more severe in the male population. Eventually, miRNA27a/b might be revealed as a biomarker for FH and its severity, but further validation studies are required in larger populations considering the impact of age, since the effect of age cannot be overlooked.