Introduction
Thyroid cancers are the most common malignancy of the endocrine system [1]. Globally, nearly 1% of all patients with newly diagnosed malignancies suffer from thyroid cancers [2]. As a subtype, papillary thyroid cancer (PTC) accounts for 80% of all thyroid cancers [3]. Even though great advances have been made in the treatment of thyroid cancer, mortality has been increasing in recent years due to it commonly being diagnosed at advanced stages [4]. Meanwhile, differentiation and distant metastasis of thyroid cancer may contribute to poor prognosis [5]. Therefore, novel therapeutic approaches and individual treatment strategies are needed [5, 6]. Consequently, there is an urgent need to explore the underlying mechanisms and develop a novel therapeutic strategy for thyroid cancer.
Long noncoding RNAs (lncRNAs) are a series of noncoding RNAs over 200 nucleotides in length [7]. It has been demonstrated that lncRNAs regulate gene expression and the eukaryotic genome in general [8]. Studies also have shown that lncRNAs play an important role in biological processes, including proliferation, apoptosis, metastasis, migration and invasion via regulating gene expression, transcription, or translation of mRNA [9, 10]. Increasing evidence has indicated the potential for lncRNAs in the treatment of human tumors, especially PTC, and revealed that lncRNAs act as modulators in various tumors [11, 12]. H19 suppresses the cell viability, migration, and invasion of thyroid cancer via regulating Bcl-2/BAX, caspase-3, and PI3K/AKT [13]. MCM3AP-AS1 contributes to the proliferation and invasion of PTC via E-cadherin pathways [14, 15]. BISPR regulates the proliferation and apoptosis of PTC cells by regulating miR-21-5p/Bcl-2 [16]. Overexpression of cancer susceptibility candidate 15 (CASC15) promotes the proliferation of cervical cancer [17].
CASC15, which is located on chromosome 6p22.3, is characterized by high activity in cancer [18]. A previous study reported that CASC15 promotes the proliferation and migration of neuroblastoma cells [19]. However, upregulated CASC15 induces the proliferation and metastasis of colon cancer cells [20]. Moreover, CASC15 contributes to epithelial to mesenchymal transition and inhibits the apoptosis of hepatocellular carcinoma cells [21]. Therefore, the roles of CASC15 in the development of cancer are controversial and elusive. Moreover, the potential roles of CASC15 in PTC have not been elucidated.
In the present study, we investigated the potential roles of CASC15 in PTC, the effects of CASC15 on the cell behavior of PTC cells, and the mechanisms driving these activities. This may provide a novel strategy for the treatment of thyroid cancer.
Material and methods
Tissues and samples
Thirty PTC tissues and adjacent normal tissues were collected from PTC patients who were enrolled in the present study and were hospitalized at the Second Hospital of Hebei Medical University from October 2016 to January 2018. None of the PTC patients received chemotherapy or radiotherapy prior to this study. All tissues were immediately stored at –80°C in liquid nitrogen until use. The study was approved and overseen by the Ethics Committee of the Second Hospital of Hebei Medical University. Meanwhile, informed consent was obtained from all patients before the study. The clinical characteristics of the patients are listed in Table I.
Table I
Expression of CASC15 and clinical characteristics
Cell culture
Papillary thyroid cancer cell lines BCPAP and K1 and immortal thyroid cell line Nthy-ori3-1 were purchased from the Institute of Biochemistry and Cell Biology of the Chinese Academy of Sciences (Shanghai, China). Cells were cultured in RPMI-1640 medium containing 10% fetal bovine serum (FBS, Wisent, Canada) and 1% penicillin/streptomycin (Invitrogen, USA) at 37°C in an atmosphere humidified with 5% CO2.
Transfection
Cells were transfected with CASC15 siRNAs and the negative control siRNA (GenePharma, Shanghai, China) with Lipofectamine 2000 (Invitrogen, USA) according to the manufacturer’s instructions. At 48 h after transfection, cells were used for the following functional assays.
CCK-8 assay
Cell counting kit-8 (CCK-8) assays (Beyotime, Shanghai, China) were used to examine the viability of PTC cells. Cells at the logarithmic growth phase were plated into 96-well plates (2 × 104 cells/well). Then, the cells were incubated at 37°C in 5% CO2 for 0, 12, 24, and 48 h. Then, the cells were treated with 10 μl of CCK-8 solution and cultured for another 2 h. The absorbance rate at 450 nm was then measured with a microplate reader (Bio-Rad Laboratories, Inc., Hercules, USA). Each experiment was performed in triplicate.
qRT-PCR
Total RNA was extracted from tissues or cells with TRIzol (Invitrogen, USA). RNA (1 μg) was reverse transcribed into cDNA using a Prime-Script RT kit (Takara, Japan) according to the manufacturer’s instructions. A SYBR Green PCR Kit (Takara, Japan) was applied to measure the mRNA levels with an ABI 7500 System. The PCRs were performed under the following conditions: 95°C for 30 s, followed by 40 cycles of 95°C for 5 s and 60°C for 30 s. The relative mRNA levels of the target genes were determined with the 2–ΔΔCt method [22]. GAPDH served as an internal control. Each experiment was performed in triplicate. The sequences of the primers used are listed in Table II.
Table II
Oligonucleotide primers used for PCR
Western blot analysis
Total protein was isolated from cells using RIPA lysis buffer (Beyotime, China). The concentration of the protein was determined with a BCA kit (Beyotime, China). Equal amounts of protein (30 μg) were separated on a 10% acrylamide gel via SDS-PAGE. Then, the protein was transferred to polyvinylidene fluoride membranes (PVDF, Beyotime, China). Later, the protein was blocked with 5% skimmed milk at 37°C for 30 min. After this, the membranes were incubated with the following primary antibodies at 4°C overnight: anti-Bcl-2 (ab32124, 1 : 1000, Abcam, USA), -E-cadherin (ab15148, 1 : 500, Abcam, USA), -AKT (ab81283, 1 : 5000, Abcam, USA), -PI3K (ab32089, 1 : 1000, Abcam, USA), and -GAPDH (ab181602, 1 : 10000, Abcam, USA). The next day, the membranes were incubated with a second anti-rabbit antibody (ab6721, 1 : 10000, Abcam, USA). The positive signals were determined with electrochemiluminescence (Thermo Fisher Scientific, Inc., USA), and the bands were captured with ChemImager 5500 V2.03 software (Alpha Innotech, CA) and analyzed with ImageJ software 1.47 (National Institute of Health, USA). GAPDH was used as an internal control.
Wound healing assay
After 48 h of transfection, cells (3 × 105 cells/well) were seeded into 24-well plates. A scratch was made with a sterile pipette (200 μl) on the bottom of the plate. Twenty-four h later, the cells were washed with PBS and then incubated with DMEM at 37°C. Subsequently, the migration of cells was calculated with an inverted microscope (200× magnification, Olympus, Tokyo, Japan). Each experiment was conducted in triplicate.
Transwell assay
Transwell chambers containing 100 μl of serum-free medium were coated with Matrigel (BD Biosciences, USA) at 37°C for 30 min. Cells (~2 × 105 cells/chamber) were harvested at 48 h after transfection and washed with PBS. Then, the lower chamber was incubated with 500 μl of serum-containing medium at 37°C for 12 h. The cells were fixed by the addition of 4% paraformaldehyde to the lower chamber; then, the cells were stained with 0.1% crystal violet for 30 min. Subsequently, the invaded cells were visualized with a Nikon light microscope (200× magnification, Nikon, Japan).
Statistical analysis
All data were analyzed with SPSS 19.0 (IBM Corporation, USA) and expressed as the mean ± SD. Student’s t test was performed to evaluate the difference between two groups. ANOVA was applied to analyze differences among multiple groups. Each independent experiment was performed three times. P < 0.05 was considered statistically significant.
Results
Expression of CASC15 in PTC tissues and cells, and the correlation between CASC15 and clinical characteristics
qRT-PCR was performed to determine the mRNA level of CASC15 in PTC tissues and cells. As shown in Figure 1 A, the mRNA level of CASC15 in PTC tissues was significantly higher than it was in adjacent normal tissues. Meanwhile, we examined the expression of CASC15 in PTC cell lines. qRT-PCR was performed to determine the mRNA level of CASC15. The expression of CASC15 was significantly increased in PTC cells (BCPAP and K1 cells) compared with Nthy-ori3-1 cells (Figure 1 B). As shown in Table I, the expression of CASC15 was significantly associated with grade (0.044) and lymphatic metastasis (0.034), while there was no significant difference between the expression of CASC15 and the age (0.996) or gender (0.691) of PTC patients. These results showed that CASC15 may be distinctively upregulated in thyroid cancer, suggesting that it might play unique roles in the progression of PTC.
Figure 1
CASC15 was upregulated in PTC tissues and cells. A – Expression of CASC15 was significantly upregulated in PTC tissues compared with adjacent normal tissues. B – Expression of CASC15 was significantly increased in BCPAP and K1 cells
**P < 0.01 vs. adjacent normal tissues. ##P < 0.01 vs. Nthy-ori 3-1. PTC – papillary thyroid cancer; CASC15 – cancer susceptibility candidate 15.
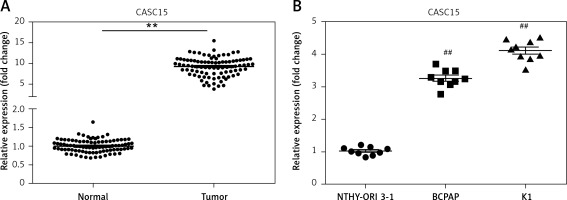
CASC15 siRNA significantly decreased the expression of CASC15
Cells were transfected with CASC15 siRNA and a negative control. The results showed that the expression of CASC15 in BCPAP cells treated with a CASC15 siRNA was significantly decreased compared with the expression in cells treated with the negative control siRNA, while there was no significant difference between the negative group and the control group (Figure 2 A). The expression of CASC15 in K1 cells mirrored the expression pattern in BCPAP cells (Figure 2 B).
Figure 2
CASC15 siRNA significantly decreased the expression of CASC15. A – After treatment with CASC15 siRNA, expression of CASC15 was significantly downregulated in BCPAP cells. B – Expression of CASC15 in K1 cells was significantly decreased after transfection with CASC15 siRNA
**P < 0.01 vs. NC group. NC – negative control, CASC15 – cancer susceptibility candidate 15.
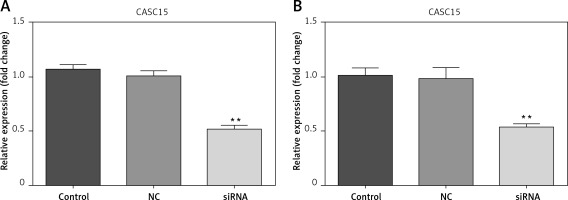
Downregulated CASC15 inhibited the viability of PTC cells
CCK-8 assays were performed to assess the proliferation of PTC cells. As shown in Figure 3 A, the proliferation of BCPAP cells was significantly decreased after treatment with the CASC15 siRNA. The difference between the control group and the NC group was not statistically significant. The BCPAP results were in line with those from K1 cells (Figure 3 B).
Figure 3
Downregulated CASC15 inhibited viability of PTC cells. A – Viability of BCPAP cells was significantly decreased after treatment with CASC15 siRNA. B – Viability of K1 cells was significantly decreased after treatment with CASC15 siRNA
**P < 0.01 vs. NC group. NC – negative control, CASC15 – cancer susceptibility candidate 15.
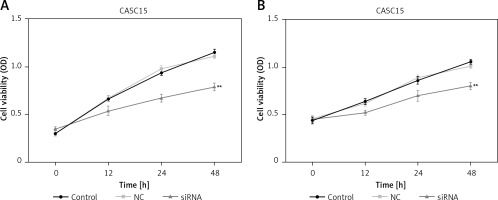
Downregulated CASC15 suppressed the migration of PTC cells
A wound healing assay was conducted to assess the migration of PTC cells. The migration of PTC cells treated with the CASC15 siRNA was significantly inhibited, while there was no significant difference between the control group and the NC group (Figures 4 A–D).
Figure 4
Downregulated CASC15 suppressed migration of PTC cells. A, B – Downregulation of CASC15 significantly inhibited migration of BCPAP cells. C, D – Downregulation of CASC15 significantly inhibited migration of K1 cells
**P < 0.01 vs. NC group. NC – negative control, CASC15 – cancer susceptibility candidate 15.
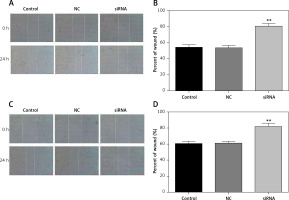
Downregulated CASC15 repressed the invasion of PTC cells
We also analyzed the invasion ability of PTC cells after treatment with the CASC15 siRNA, as shown in Figures 5 A–D. The results showed that the invasion rate of the cells treated with the CASC15 siRNA was significantly lower than it was in the NC group. The difference between the control group and the NC group was not significant.
Figure 5
Downregulated CASC15 repressed invasion of PTC cells. A, B – Downregulation of CASC15 significantly inhibited BCPAP cell invasion. C, D – Downregulation of CASC15 significantly inhibited invasion of K1 cells
**P < 0.01 vs. NC group. NC – negative control, CASC15 – cancer susceptibility candidate 15.
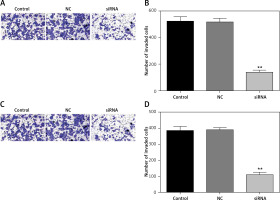
CASC15 regulated the mRNA levels of Bcl-2/BAX, E-cadherin, caspase-3, and PI3K/AKT
qRT-PCR experiments were performed to examine the mRNA levels of Bcl-2/BAX, E-cadherin, and caspase-3. The results showed that downregulated CASC15 significantly decreased the mRNA levels of Bcl-2 and PI3K/AKT and increased the mRNA levels of BAX, E-cadherin and caspase-3 (Figure 6 A–L).
Figure 6
CASC15 regulated mRNA levels of BAX, E-cadherin, Bcl-2 and caspase-3. A–F – Downregulation of CASC15 significantly decreased mRNA levels of Bcl-2, and PI3K/AKT and increased mRNA levels of E-cadherin, caspase-3, and BAX in BCPAP cells. G–L – Downregulation of CASC15 significantly decreased mRNA levels of Bcl-2 and PI3K/AKT, and increased mRNA levels of E-cadherin and caspase-3 in K1 cells
**P < 0.01 vs. NC group. NC – negative control, CASC15 – cancer susceptibility candidate 15.
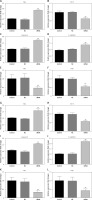
CASC15 regulated the protein levels of PI3K/AKT, Bcl-2/BAX, E-cadherin, and caspase-3
Western blot experiments were performed to determine the protein levels of PI3K/AKT Bcl-2/BAX, E-cadherin, and caspase-3. As shown in Figures 7 A and B, the protein level of PI3K/AKT showed no significant difference between the groups, and the protein level of Bcl-2 was significantly decreased in BCPAP and K1 cells following treatment with the CASC15 siRNA; however, Bax, E-cadherin and caspase-3 were significantly increased after the treatment. There was no significant difference between the control group and the NC group.
Figure 7
CASC15 regulated protein levels of PI3K/AKT, E-cadherin, Bcl-2 and caspase-3. A – Decreased CASC15 downregulated the protein level of Bcl-2 and increased the protein levels of BAX, caspase-3, and E-cadherin in BCPAP cells. The protein level of PI3K/AKT showed no significant changes. B – Decreased CASC15 downregulated the protein level of Bcl-2 and increased the protein levels of BAX, caspase-3, and E-cadherin in K1 cells. The protein level of PI3K/AKT showed no significant changes
**P < 0.01 vs. NC group. NC – negative control, CASC15 – cancer susceptibility candidate 15.
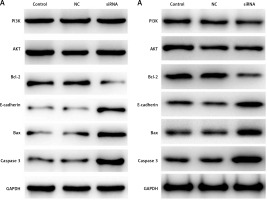
Discussion
Papillary thyroid carcinoma ranks as the most common malignancy seen in clinical practice. Various factors complicate PTC therapy. Past decades have witnessed advances in research on the molecular mechanisms directing pathogenesis. Zara-Lopes et al. demonstrated that MTHFR, MTR, RFC1 and CβS are involved in the progression of PTC [22]. In recent years, increasing attention has focused on the potential roles of lncRNAs in cancer development and progression. Dysregulated lncRNAs in PTC have been verified; however, the biological function and underlying mechanisms of many PTC-associated lncRNAs remain largely unknown. In this study, we found that CASC15 was upregulated in PTC tissues and cells. Moreover, the abnormally expressed CASC15 was significantly correlated with tumor grade and lymphatic metastasis. These results showed that CASC15 may act as an oncogene in PTC. However, the underlying mechanisms remain unclear.
Previous studies revealed that lncRNAs play crucial roles in regulating the tumor processes of PTC, such as proliferation, apoptosis, migration and invasion [11–15]. However, the roles of CASC15 in cancer are controversial and elusive. CASC15 acts as an oncogene in breast cancer as well as a tumor suppressor in neuroblastoma [18, 23]. To further elucidate the underlying mechanisms of CASC15 in PTC, we investigated the potential effects of CASC15 on the cell proliferation, migration and invasion of PTC cells. lncRNAs are involved in the tumorigenesis and development of PTC via regulation of proliferation, apoptosis, migration and invasion [24, 25]. Downregulation of the lncRNA PVT1 suppresses the proliferation of PTC cells and blocks the cell cycle at the G0/G1 stage [26]. Upregulated MEG3 inhibits the migration and invasion of PTC cells [27]. In the present study, the results showed that downregulated CASC15 inhibited the proliferation, migration and invasion of PTC cells, which further demonstrated that CASC15 is an oncogene in PTC and that knockdown of CASC15 may play a positive role in regulating the migration and invasion of PTC cells. These results are consistent with those reported in a study by Yu et al., which revealed that CASC15 acts as an oncogene and contributes to the progression of breast cancer cells [23]. However, the underlying molecular mechanisms remain unknown.
The PI3K/AKT pathway plays an important role in cell proliferation, EMT, the cell cycle, and apoptosis in PTC [13]. BCL-2 plays a vital role in oncogenesis in cancer [28]. Caspase-3 plays an important role in the progression of cancers. Activation of E-cadherin is associated with the migration and invasion of PTC [29]. Furthermore, CASC15 regulates the proliferation, apoptosis, migration and invasion of gastric cancer and hepatocellular carcinoma via BCL-2/BAX, caspase-3, and E-cadherin [30, 31]. In the present study, knockdown of CASC15 suppressed the expression of Bcl-2 and increased the expression of caspase-3 and E-cadherin. These results showed that the regulatory roles of CASC15 in the PI3K/AKT, BCL-2/BAX, caspase-3 and E-cadherin pathways may inhibit the progression of PTC, which suggested that downregulated CASC15 inhibited the proliferation, migration and invasion of PTC cells.
While this study is the first to elucidate the potential roles of CASC15 in PTC, it is limited by the number of patients and lack of in vivo assays. CASC15 may regulate the BCL-2/BAX, caspase-3 and E-cadherin pathways via sponging microRNA(s). This requires further study.
In conclusion, CASC15 was upregulated in PTC tissues and cells. CASC15 may regulate the proliferation and invasion of PTC cells through the PI3K/AKT, caspase-3 and E-cadherin pathways. These insights may provide a novel strategy for the treatment of PTC.