Introduction
Sepsis is a serious problem having increasing incidence globally [1]. Sepsis is involved in infection and inflammation; it can lead to multiple organ failure and death [2, 3]. The mortality rate remains high despite having a number of treatment approaches for sepsis [4]. Hence, there is an urgent need for developing new and effective treatment approaches for treating sepsis.
Acute respiratory distress syndrome (ARDS) is one of the dangerous forms of acute lung injury characterized by an inflammatory response of the respiratory system. ARDS leads to respiratory failure along with damage to the parenchyma of lungs [5, 6]. Both ARDS and acute lung injury are associated with sepsis, making it as a major risk factor [7]. Lungs are the most vulnerable to sepsis and hence contribute primarily to deaths in cases of respiratory failure [8]. It is reported that patients diagnosed with sepsis-mediated ARDS have high severity for illness which also increases the rate of mortality [5]. The mechanisms involved in ARDS and ALI include higher infiltration rates of proteins, leucocytes, inflammation and elevated oxidative stress [9]. Therapies improving pathologic conditions of ARDS would be beneficial [10].
Sirtuins (SIRT) have been reported to show diverse roles in various physiological processes including heart disease, neurodegenerative disorders, cancer and inflammation [11]. There are 7 members of the SIRT family (SIRT1-SIRT-7); these SIRT members vary in structure, functions and localization. Among all the members SIRT1 is the most widely studied [12, 13]. SIRT1 is found to regulate the expression of inflammatory mediators in lungs of cigarette smokers. SIRT1 regulates the expression of nuclear factor κB (NF-κB) and p65 in both in vitro and in vivo models; it regulates acetylation of NF-κB, which is crucial for the release of proinflammatory mediators [14].
MicroRNAs are small non-coding RNAs, responsible for suppression of the transcription and translation process via binding directly to the target site and can regulate gene expression [15]. MicroRNAs play a potential role in regulating the cellular process, differentiation, metabolism, proliferation and apoptosis [16, 17]. MiRNAs have also been identified to play a potential role in pulmonary fibrosis, lung cancer, tuberculosis, and bronchial hypersensitivity. MiR-133a-3p belongs to the miR-133 family of miRs. MiR-133a-3p occurs in the human genome with different copies such as miR-133a-1, -2 and is present on the 18th and 20th chromosome [18]. To date multiple functions of miR-133a-3p have been established such as regulation of myoblasts [19], inhibition of proliferation of cardiomyocytes [20], in malignancies such as lung cancer [21], colon cancer [22] and bladder cancer [23]. Recently miR-133a-3p along with 155-5p has been profiled in diagnosis and prognosis of sepsis [24].
In a study recently involving differentiation of cardiomyocytes in developing heart, it was evidenced that the 3′UTR region of SIRT1 had a potential binding site for miR-133a, and the binding of miR-133a regulated the expression of SIRT1. The report ultimately confirmed that expression of SIRT1 is regulated by miR-133a [25]. However, the role of miR-133a-3p in sepsis-mediated ARDS has not been studied. The present study evaluated the role of miR-133a in sepsis-induced ARDS and also confirmed the pathway involved.
Material and methods
Animals and ethics statement
For the study adult male C57BL/6 mice aged between 6 and 8 weeks were used and procured from Jackson Laboratory USA. All the experimental protocols were in agreement with ethical guidelines from the Ministry of Health, China. The protocols were approved by the animal ethical committee of China-Japan Friendship Hospital, Beijing, China. The animals were housed under standard room temperature conditions (24–25°C) with a 12-hour light and dark cycle. The animals were fed with water and pallet diet ad libitum.
Histological examination
At the end of the study the mice were sacrificed and the lung specimens were isolated. The lung tissues were fixed in paraffin and sections of 5 µm were obtained using a rotary microtome. The sections of lung tissues were stained with hematoxylin and eosin.
Western blotting analysis
Part of lung tissues were used for histological studies and the remains were used further for studying the expression of proteins. The lung tissues were homogenized in a tissue homogenizer using PBS as vehicle and then centrifuged; the supernatants were used for expression of proteins. To evaluate the protein levels of Bcl-2, cleaved caspase-3 and Bax, western blot analysis was done. About 50 µg of protein was submitted to SDS-PAGE followed by transfer to PVDF membranes. The membranes were further incubated at 4ºC for 12 h along with Iry antibodies specific to Bcl-2 (1 : 1000, Abcam, USA), Bax (1 : 1000, Abcam, USA), cleaved caspase-3 (1 : 1000, Cell Signaling Tech., USA), β-actin (1 : 1000, Cell Signaling Tech., USA), SIRT1 (1 : 1000, Abcam, USA). The membranes were incubated with horseradish peroxidase secondary antibodies (1 : 3000) for 60 min. The bands of protein obtained were viewed using the ECL Western Blotting Substrate kit (Abcam USA). Densitometric analysis was done for expression of target proteins against the loading control.
RT-PCR analysis
For RT-PCR study, 500 ng of RNA was extracted from the isolated lung tissues using an RNA isolation kit (Sigma Aldrich USA). The isolated RNA was subjected to reverse transcription using the TonkBio RT reagent Kit (TonkBio, USA). The cDNAs were processed and amplified using Teloprime full length cDNA amplification kit V2 (Lexogen Austria). The study was done using a real-time system, Bio-Rad USA. The PCR conditions were as follows: denaturation of proteins for 30 s at 95°C, normalization for 10 s at 60°C, followed by elongation for 20 s at 72°C; about 30 cycles were done for the whole processing. The expression of mRNA was normalized against miRNA levels of U6 and mRNA levels of GAPDH. The sequences of primers used are described in Table I.
Table I
Primer sequences used for the study
Myeloperoxidase activity
For studying the effects of inflammation in lungs, MPO activity was assessed. The animals were sacrificed and the lung tissues were harvested; the lungs were weighed and stored at –80°C. The lung tissues were analyzed for myeloperoxidase (MPO) using an MPO activity assay kit (Abcam, USA) following the supplied instructions.
Infiltration of Evan′s blue dye
The quantification of albumin was done by measuring the albumin conjugated with Evan′s blue (Sigma Aldrich USA). Infiltration of Evan′s blue dye (20 mg/kg) was done by injecting it via the retro-orbital plexus of mice. After injecting, the mice were rested for 60 min and were then sacrificed. The lungs were subjected to perfusion using phosphate buffer saline through the right ventricle, removed and weighed and were then homogenized in a tissue homogenizer in 500 µl of formaldehyde. The homogenates were incubated at 37°C for 24 h followed by centrifugation at 10000 rpm for 20 min. The supernatants were collected and absorbance was recorded at 620 nm. The quantification of Evans blue dye in lungs was done by creating a standard calibration curve.
Isolation of alveolar macrophages from lungs
The alveolar macrophages were separated from the lungs of mice by a bronchoalveolar lavage procedure, counted and were suspended again in DMEM/F-12 medium (Thermo Fisher, USA) pre-supplemented with fetal bovine serum (10%), penicillin (100 U/ml) and streptomycin (100 U/ml) (Sigma Aldrich USA). The isolated alveolar macrophages were submitted to sepsis challenge in vitro, in which they were treated with lipopolysaccharide (LPS) (1 µg/ml) (Sigma Aldrich USA) for 4 h. Before inducing the macrophages, the medium was added with SIRT1 and EX527 (1 µM) 24 h prior to LPS stimulation.
Development of burn sepsis model in mice
For the in vivo study, C57BL/6 mice aged 6 to 8 weeks were used and were anesthetized with injection of sodium pentobarbital (50 mg/kg); the back area of mice were shaved to remove the hairs. The shaved back area of 2 cm2 was exposed to steam for 8 s for burning the skin for sepsis. The burned back region was cooled at room temperature for 5 min; the surface area was incubated with a suspension of Pseudomonas aeruginosa bacteria (50 µl) using a microinjector. Immediately after the burning, the mice were injected with normal saline via the intraperitoneal route (1 ml/20 g). In the process of establishing the burn sepsis model, miR-133a-3p antagomir (80 mg/kg/day) was injected into the caudal vein for 3 continuous days. For inhibition, the mice were injected with normal saline, SIRT1 or EX527 (5 mg/kg).
Enzyme-linked immunosorbent assay
After 24 h of LPS stimulation, the mice were subjected to anesthesia using pentobarbital injection (30 mg/kg). Blood was collected and serum was extracted followed by analysis of tumor necrosis factor-α (TNF-α) using an ELISA kit (Thermo Fisher USA).
Transfection of macrophages
For the purpose of transfecting the cells, miR-133a-3p agomir or miR-133a-3p antagomir and their respective control RNAs were used (Qiagen). The macrophagic cells received transfection of scrambled negative control miR-133a-3p, miR-133a-3p antagomir or agomir using a transfecting reagent (X-tremegene, Sigma Aldrich USA). Briefly, about 20 µM of miR-133a-3p antagomir or agomir was added and mixed with 12 µl of transfection reagent in about 100 µl of culture medium for 10 min at room temperature conditions to obtain the transfection complex. The macrophages were incubated along with complexes for 48 h.
Luciferase assay
The 3′UTR region fragment of SIRT1 possessing the binding site for miRNA was amplified with the help of PCR; the cDNA template obtained from the macrophage RNA was used for the same. The mutant 3′UTR and the wild type 3′UTR having the potential binding sites for the sequence of miR-133a-3p were cloned downstream of the firefly luciferase gene in the pGL3 vector. The cells received co-transfection of miR-133a-3p or control miRNA. After 48 h from transfection, the cells were washed in phosphate buffer saline and the luciferase activity was measured with the help of the dual luciferase reporter assay system.
Results
LPS stimulation increases the level of miR-133a-3p in macrophages
LPS is identified to be one of the main factors in induction of acute lung injury mediated ARDS with alveolar macrophages being the main target cells. The results of RT-PCR suggested that the mRNA levels of TNF-α, interleukin 6 (IL-6) and IL-1β were upregulated significantly in macrophages when stimulated with LPS (100 ng/ml) compared to the control (Figures 1 A–C). The miR-133a-3p levels were also upregulated significantly after LPS treatment (Figure 1 D). The findings of the experiment suggested a potential role of miR-133a-3p in LPS-mediated upregulation of inflammatory cytokines in macrophages.
Figure 1
Levels of proinflammatory cytokines and miR-133a-3p in alveolar macrophages after stimulation with LPS. A–C – RT-PCR evaluation showing relative mRNA levels of IL-1β, IL-6 and TNF-α in alveolar macrophages after stimulation with LPS. D – miR-133a-3p levels in alveolar macrophages after stimulation with LPS
The data presented show means ± SE (n = 6); **p = 0.01 versus control.
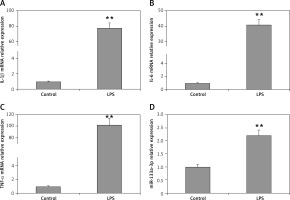
miR-133a-3p is essential for LPS-mediated upregulation of inflammatory cytokines in macrophages
To evaluate the role of miR-133a-3p in LPS-mediated upregulation of inflammatory cytokines, the alveolar macrophages were transfected with miR-133a-3p antagomir or agomir, so as to increase or decrease the expression levels of miR-133a-3p. As presented in Figure 2 A, the mRNA levels of IL-1β in macrophages increased significantly after treatment of LPS. The outcomes also suggested that the pre-exposure of miR-133a-3p antagomir decreased the mRNA levels significantly. It was also found that the mRNA levels of IL-6 and TNF-α decreased significantly with a decrease in levels of miR-133a-3p (Figures 2 B, C). Furthermore, it was evidenced that, when the levels of miR-133a-3p were upregulated, it resulted in increased mRNA levels of TNF-α, IL-6 and IL-1β, as shown in Figures 2 D and F. Altogether, the results of the experiment confirmed that miR-133a-3p is crucial for LPS-mediated upregulation of inflammatory cytokines in macrophages.
Figure 2
Results of miR-133a-3p treatment on LPS-mediated over-expression of inflammatory cytokines in alveolar macrophages. A–C – RT-PCR evaluation demonstrating the mRNA expression levels of IL-1β, TNF-α and IL-6 in alveolar macrophages in presence and absence of miR-133a-3p antagomir after LPS stimulation. D–F – Results of RT-PCR demonstrating the mRNA levels of IL-1β, TNF-α and IL-6 in alveolar macrophages in presence and absence of miR-133a-3p agomir after LPS stimulation
The data presented show means ± SE (n = 6); **p = 0.01 versus control. (NC) negative control.
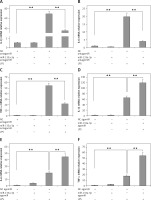
Downregulated miR-133a-3p plays a protective role in mouse ARDS
The experiments so far have confirmed that miR-133a-3p is essential in upregulation of LPS-mediated pro-inflammatory cytokine. In the study expression of miR-133a-3p in the mouse model of sepsis was suppressed to study the role of miR-133a-3p in lung injury. The results (Figure 3 A) demonstrated that treatment of miR-133a-3p antagomir in C57BL/6 mice significantly decreased lung congestion, edema and hemorrhage compared to the negative control and antagomir group. The results of ELISA suggested that the expression of TNF-α, which is a pro-inflammatory factor, increased significantly in lung tissues of sepsis-induced mice compared to the sham group, and the miR-133a-3p antagomir reversed the elevated levels of TNF-α (Figure 3 B). The MPO activity was measured for assessing the activity of neutrophils in the lungs. The results are shown in Figure 3 C; the treatment of miR-133a-3p antagomir decreased the elevated MPO activity significantly in lungs of mouse with sepsis. The infiltration study of Evan′s blue dye suggested that the treatment of miR-133a-3p antagomir decreased the protein leakage in lungs of sepsis-induced mice (Figure 3 D). The protein levels of Bax and cleaved caspase-3 were elevated significantly in the lung tissues of sepsis-induced mice; the treatment of miR-133a-3p antagomir decreased the levels of protein significantly (Figure 3 E). The experiments also confirmed decreased levels of Bcl-2 protein in sepsis-induced lung tissues; the treatment of miR-133a-3p antagomir increased the levels of Bcl-2 in lungs of sepsis-induced mice (Figure 3 E). These findings suggest an attenuating role of miR-133a-3p in septic mice.
Figure 3
Results of miR133a-3p in acute sepsis-induced acute respiratory distress syndrome. A – Results of hematoxylin and eosin (H&E) staining depicting the morphological changes in sepsis-induced lung tissue after 24 h of injury. The negative control miR-133a-3p antagomir exposed sepsis injury group (NC antagomir) exhibited increase in lung edema, hemorrhage in alveoli, infiltration of neutrophils and damaged epithelial/endothelial cell structure compared to the sham operated group. miR-133a-3p antagomir exposed sepsis animals (miR-133a-3p antagomir) exhibited significant attenuation in morphology of lung tissues. Scale bar, 50 μm. B – ELISA study exhibiting the effect of miR-133a-3p antagomir on levels of TNF-α in sepsis-induced lung injury after 24 h of treatment. C – Activity of MPO in sepsis-induced lung injury 24 h after miR-133a-3p antagomir treatment in every treatment group was recorded. D – Levels of Evan′s blue dye evaluated in each group and treatment of miR-133a-3p antagomir caused a significant reversal and elevated the levels of Evan′s blue dye mediated by sepsis injury after 24 h of treatment. E – Western blot analysis exhibiting the effect of miR-133a-3p on expression levels of protein, i.e. cleaved caspase-3, Bcl-2 and Bax in sepsis injury lung tissues after 24 h of treatment. F – Quantitative analysis results for relative expression of proteins C-caspase, Bcl-2 and Bax
Data are presented as means ± SE (n = 6); **p < 0.01 compared to sham operated group.
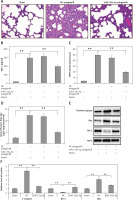
SIRT1 is a direct target of miR-133a-3p in macrophages
MicroRNAs have been found to function mainly by regulating the target genes. With the possibility to identify the target genes of miR-133a-3p in alveolar macrophages, gene databases such as miRanda, TargetScan and PicTar were explored. The databases suggested that the SIRT1 gene had a favorable target site for miR-133a-3p in the 3′-UTR region. The database showed a match between the target site and the seed region of miR-133a-3p (Figure 4 A). As demonstrated, the luciferase activity was decreased significantly by miR-133a-3p mimics in the 3′-UTR of wild type, but the activity remained unsuppressed in the presence of the reporter transfected with mutant 3′-UTR, suggesting direct binding of miR-133a-3p to the 3′-UTR region of SIRT1 (Figure 4 B). Also it was evidenced that upregulation of miR-133a-3p in macrophages suppressed the expression of SIRT1 at protein as well as at mRNA levels, whereas the inhibition of miR-133a-3p elevated the expression of SIRT1 (Figures 4 C, D). These findings suggested SIRT1 as a favorable target of miR-133a-3p which inhibits its levels.
Figure 4
SIRT1 is a potential target for miR-133a-3p. A – The 3′UTR region of SIRT1 having a binding sequence with miR-133a-3p. Bioinformatics analysis identifies the interaction of SIRT1 with miR-133a-3p. B – The cells were cultured and received transfection with wild-type (WT) or mutated (MUT) SIRT1 3′UTR constructs of luciferase reporter followed by miR-133a-3p mimics for 48 h and then submitted to luciferase analysis. C – Immunoblotting and qRT-PCR analysis for expression of SIRT1 in alveolar macrophages which were transfected with negative control and agomir-133a-3p. D – qRT-PCR and Western blot evaluation of SIRT1 levels in alveolar macrophages transfected with negative control and miR-133a-3p antagomir
Data are presented as means ± SE (n = 6). **P < 0.01 compared to negative control.
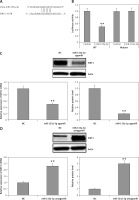
Inhibition of SIRT1 halts the protective effect of miR-133a-3p antagomir
Outcomes of the study until now have confirmed that SIRT1 is a favorable target of miR-133a-3p. To confirm this, a SIRT1 inhibitor (EX527) was used to suppress the activity of SIRT1 and its role in upregulation of inflammatory cytokine in LPS-mediated alveolar macrophages. The results showed that (Figure 5 A) the decreased mRNA levels of IL-1β in macrophages after LPS treatment were attenuated significantly by the SIRT1 inhibitor EX527 (Figure 5 A), which also corrected the decreased mRNA levels of TNF-α and IL-6 (Figures 5 B, C). These findings confirm that suppression of SIRT1 is essential in LPS-induced lung injury in alveolar macrophages through miR-133a-3p and also SIRT1 could play an important protective role.
Figure 5
The SIRT1 inhibitor EX-527 inhibits the protective role of miR-133a-3p antagomir. A–C – Results of qRT-PCR analysis demonstrating the mRNA levels of IL-1β, IL-6 and TNF-α in alveolar macrophages in presence and in absence of EX-527 after LPS + miR-133a-3p antagomir treatment
Data are presented as means ± SE (n = 6). **P < 0.01 compared to group devoid of EX-527 treatment.
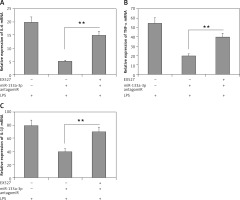
The attenuating effect of miR-133a-3p antagomir in sepsis-induced lung is SIRT1 dependent
The findings have confirmed that decreased miR-133a-3p levels play an attenuating role in septic lungs in mice. The study further evaluated whether the protective role of SIRT1 is via upregulation of SIRT1 levels or not. For this, a SIRT1 inhibitor in sepsis-induced ARDS mice was used. It was found that treatment with miR-133a-3p antagomir + EX527 in mice aggravated the edema and lung congestion more significantly compared to the miR-133a-3p antagomir group (Figure 6 A). The levels of TNF-α were elevated significantly after receiving treatment with EX527 (Figure 6 B). The results of MPO activity and protein leakage increased significantly after receiving the EX527 treatment (Figures 6 C, D). The EX527 treatment reduced the levels of Bax of caspase-3 and increased Bcl-2 after the macrophages were treated with miR-133a-3p antagomir (Figure 6 E). The results hence suggest that miR-133a-3p exerts an attenuating role in the mouse sepsis model via upregulating the level of SIRT1.
Figure 6
Effects of SIRT1 inhibitor EX-527 on the protective effect of miR-133a-3p antagomir in sepsis-induced lung injury. A – H&E demonstrating the changes in morphology of the lung tissue induced by sepsis injury after 24 h of injury. The negative control miR-133a-3p antagomir sepsis injury group (NC-antagomir) demonstrated increase in lung edema, hemorrhage of alveoli, infiltration of neutrophils and damaged epithelial/endothelial cell structure compared to the sham operated group. The miR-133a-3p antagomir sepsis injury group (miR-133a-3p antagomir) demonstrated substantial attenuation on morphology of lung tissues, whereas the SIRT1 inhibitor EX-527 (miR-133a-3p antagomir + EX-527) substantially inverted this improvement. (Scale – 50 μm) B – ELISA study demonstrated the effects of EX-527 treatment on the expression of TNF-α in sepsis-induced lung injury. C – Activities of MPO in sepsis injured lung tissues after 24 h of treatment of miR-133a-3p antagomir in presence or absence of without EX-527 in every group. D – Levels of Evan′s blue dye were studied in each of the three; the results suggested that EX-527 reversed the decrease in levels of Evan′s blue dye mediated by miR-133a-3p antagomir. E – Western blot analysis for demonstrating the effect of EX-527 on protein levels of cleaved caspase-3, Bax, and Bcl-2 in sepsis injured lung tissues after 24 h of treatment
The results are means ± SE (n = 6). **P < 0.01.
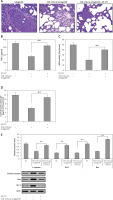
Discussion
Sepsis is one of the most common factors in ALI/ARDS. It occurs due to a favorable interaction between invading microbes and the immune system of the host, producing an acute inflammatory as well as coagulation reaction [4, 26, 27]. Sepsis is accompanied by a serious systemic inflammatory response, which leads to damage of vital organs [28]. ARDS is a state showing acute respiratory failure and is characterized by diffusive infiltration and hypoxemia [29]. Studies have reported that the rate of sepsis-mediated mortality in subjects with ALI/ARDS has declined in the last 10 years but still the rate remains near to 40%, which is still critical [30, 31]. In sepsis-mediated lung injury, a number of inflammatory cytokines are liberated in the extracellular and intracellular matrix which leads to damage of alveolar membrane and dysfunctioning of alveolar capillary diffusion, leading to hypoxemia [19, 32]. Previous studies have demonstrated that migration and activation of the monocyte macrophage system cause over-release of inflammatory mediators such as TNF-α, IL-6 and IL-1β, which are reported to be significant inducers of tissue injury and dysfunction [33–35].
Growing studies have shown that some specific genes play a vital role in the prognosis and pathogenesis of ARDS and sepsis. Interestingly, miRs have attracted major attention of researchers as being possible therapeutic targets for halting the progression of ARDS [16, 36]. miR-133a-3p belongs to the miR-133 family of miRs; it is a multifunctional mRNA expressed in a number of pathologic conditions. The miR-133 family of miRs has been found to regulate the proinflammatory factors [37]. Production of proinflammatory cytokines is important for sepsis-mediated ARDS. It is found that both TNF-α and IL-1β are upregulated in patients reported for sepsis. Targeting TNF-α and IL-1β decreased the development of sepsis and also improved the survival rate in both animal models as well as in patients [5, 38]. In the present work, a miR-133a-3p antagomir decreased the levels of proinflammatory cytokines in macrophages and sepsis-induced lung tissues, indicating that miR-133a-3p antagomir may exert a protective effect on lungs via modulating the expression of proinflammatory mediators. It was also evidenced that the treatment of miR-133a-3p antagomir suppressed the activity of MPO in lung tissues, which is an important biomarker for activation of neutrophils [39]. These findings suggest that miR-133a-3p antagomir may modulate infiltration of inflammatory cells. It may be possible that miR-133a-3p can target polymorphonuclear neutrophils so as to decrease the MPO activity, which needs to be studied further. In the present study, the decreased infiltration of Evan′s blue dye in the sepsis-induced lungs demonstrated decreased permeability of microvasculature of endothelium and also attenuated its integrity. These findings suggest that downregulated miR-133a-3p shows a proinflammatory effect in sepsis-mediated ARDS. There is increasing evidence suggesting an increase in epithelial or endothelial cell apoptosis in the development of ARDS [32, 40]. It has already been established that ARDS is accompanied by increased cell death, but the apoptotic inhibitors elevate the cell survival significantly in the sepsis model of ARDS [41, 42]. Bcl-2, caspase-3 and Bax have been identified to play important roles in apoptotic cell death [43]; the findings were in agreement with this study and suggested a significant decrease in levels of caspase-3 and Bax in the lung tissues after treatment with miR-133a-3p antagomir. These results confirmed the apoptosis inhibitory activity of miR-133a-3p antagomir. Overall, these findings confirmed that miR-133a-3p antagomir exerts an attenuating effect in ARDS and can be an important therapeutic target for treating ARDS.
Sirtuin-1 is also called NAD-dependent deacetylase sirtuin-1; it is a protein that in humans is converted by the SIRT1 gene. It is associated with cell growth, cell differentiation, metabolism and decrease of oxidative burden [44, 45]. SIRT1 is reported to be responsible for anti-oxidative and anti-inflammatory effects, modulating apoptosis and inhibiting DNA damage in different types of cells. Reports have suggested a role of SIRT1 in relation to macrophages [46, 47]. In a study recently, SIRT1 was found to play an attenuating role in burn-induced ARDS [48]. In the present study, it was demonstrated that SIRT1 is one of the favorable targets of miR-133a-3p; this finding was in agreement with a study in which SIRT1 was regulated by miR133a-3p and was confirmed to have potential binding sites for it [25]. In addition, pre-exposure of LPS-induced alveolar macrophages to SIRT1 inhibitor resulted in reversal of the anti-inflammatory effect of miR133a-3p antagomir and also reversed the protective effect of miR-133a-3p antagomir in a sepsis-mediated ARDS model. The findings hence suggested that miR-133a-3p regulates the sepsis-mediated ARDS via degradation of SIRT1. Altogether, the outcomes of bioinformatics and luciferase assay confirm that SIRT1 is a potential target gene of miR-133a-3p in sepsis-mediated ARDS.
The outcomes of the present work may be helpful for designing therapy for sepsis-induced ARDS. As it was evidenced that SIRT1 was a potential target, it would facilitate more efficient treatment of ARDS.
In conclusion, it was demonstrated for the first time that miR-133a-3p induces the expression of proinflammatory factors in a sepsis model of ARDS, and the protective role of miR-133a-3p antagomir in ARDS is due to its attenuating effect on secretion of inflammatory cytokines and inhibition of SIRT1-mediated apoptosis. These findings will definitely improve the knowledge about the mechanism involved in the regulatory effect of miR-133a-3p in sepsis-induced acute lung injury and will also guide us in developing new therapeutic agents for targeting miR-133a-3p.