Introduction
The recent outbreak of coronavirus disease 19 (COVID-19), an infection caused by the virus SARS-CoV-2, in Wuhan, China, has caused devastating repercussions worldwide [1–3]. The epidemic spread rapidly, and on March 11, 2020 it was characterized as a pandemic by the World Health Organization (WHO). Until August 23rd 2020, there have been 23 546 173 cases, and 811 436 deaths worldwide [4]. In the attempt to stop this pandemic, especially in severe cases, there have been several interventions evaluated in randomized controlled trials for the treatment of COVID-19 [2, 4, 5].
Convalescent plasma (CP) therapy is a classic and old immunotherapy, whose main determinant of efficacy is its neutralizing activity. It also is supposed to have antiviral activity based on the large reductions of the viral loads in a case series [6]. According to a case series of 13 patients with Middle East respiratory syndrome coronavirus (MERS-CoV), for an effective infusion of CP, donor plasma should have at least the neutralization activity of a plaque-reduction neutralization test (PRNT) titer of 1 : 80 [7].
There are several studies, most of them observational and a few randomized controlled trials (RCTs), evaluating CP efficacy and safety in several infectious outbreaks. Unfortunately, there are still no good quality systematic reviews and meta-analyses of RCTs in COVID-19 patients. In a case series of 80 patients with severe acute respiratory syndrome coronavirus (SARS-CoV) in 2003, the authors reported a higher 22-day hospital discharge rate in those 42 patients who received CP within the first 14 days of disease vs. those 38 patients who received CP after 14 days of disease (58.3% vs. 15.6%), and a reduction in the mortality rate (6.3% vs. 21.9%), with no adverse events reported [8]. In a matched controlled prospective cohort among 93 patients with severe pandemic influenza A (H1N1) 2009 virus infection, the CP group had a reduced mortality rate in comparison to those not receiving CP (OR = 0.20, 95% CI: 0.06–0.69) [9]. However, adverse events such as transfusion-related acute lung injury (TRALI) and transfusion-related circulation overload (TACO) raise concern since data are scarce [10].
On August 23rd 2020, the US Food and Drug Administration (FDA) issued an Emergency Use Authorization (EUA) for the use of CP as a treatment of COVID-19 in hospitalized patients [11]. This decision was based on results of a recent case series study that claimed that 7-day mortality was reduced by 35% in relative terms in 515 recipients of high IgG CP in comparison to 561 recipients of low IgG CP (7-day mortality: 8.9% vs. 13.7%, respectively) [12]. On September 1st 2020, the COVID-19 Treatment Guidelines Panel stated there were insufficient data to recommend either for or against the use of CP and it should not be considered standard of care [13].
In the need of more evidence-based practice guidelines for the treatment of severe COVID-19, the aim of this systematic review and meta-analysis was to evaluate the efficacy and safety of CP in human studies for the treatment of severely ill COVID-19 patients.
Material and methods
Search strategy and selection criteria
We performed a systematic review of RCTs and observational studies evaluating the effects of CP in hospitalized, confirmed COVID-19, adult patients. We included RCTs, case series, and cohorts. We excluded studies with patients < 18 years old, pregnant patients, and hepatitis B or HIV coinfection, narrative reviews, editorials, and letters to the editor.
Searches were conducted until November 24, 2020 in 5 engines: PubMed, Web of Science, Scopus, Embase and the Cochrane Library; pre-prints on medRxiv.org, and ongoing RCTs on: www.Clinical-Trials.gov, www.who.int/ictrp/about/en/, and www.clinicaltrialsregister.eu/. Search strategies were adjusted for each engine using the following combination of keywords: “convalescent plasma” AND (“COVID-19” OR “coronavirus” OR “coronavirus disease” OR “coronavirus disease-19” OR “severe acute respiratory syndrome” OR “SARS-CoV-2”) with no limitations for time or language. Included studies specified at least one efficacy or harm outcome. PubMed strategy is included in the Supplementary material (Supplementary Appendix S1).
Three reviewers (VP, AP, LNS) collected records in www.myendnoteweb.com. Two independent reviewers (APdR, RCV) assessed titles and abstracts for eligibility according to the inclusion and exclusion criteria. Discrepancies were resolved by discussion. Three independent reviewers (LNS, APdR, RCV) assessed full-text articles and extracted data with disagreements resolved by a third reviewer (AP). Extracted information included: study authors, year of publication, study design, number of patients, country, median age, proportion of males, comorbidities (obesity, hypertension, diabetes, coronary artery disease (CAD), chronic kidney disease (CKD), chronic obstructive pulmonary disease (COPD)), PCR method for COVID-19 diagnosis, CP dose and duration, concomitant treatments for both arms, primary outcomes per arm, and secondary outcomes per arm.
Primary outcomes were all-cause mortality, and clinical improvement or recovery (e.g. 2-point reduction in a 6-point ordinal severity scale or other definitions). Secondary outcomes were overall and specific adverse events, serious adverse events (SAEs), need for invasive ventilation, length of hospital stay, and treatment discontinuation.
Data analysis
Assessment of risk of bias was performed independently by 3 investigators (AP, APdR, RCV) using the Cochrane RoB 2.0 tool [14] for RCTs, and the ROBINS-I tool [15] for cohort studies with a third reviewer (AVH) resolving discrepancies when needed.
We reported our systematic review according to 2009 PRISMA guidelines [16]. Effects of CP on outcomes from individual studies were reported as hazard ratio (HR) or absolute risk difference (ARD) or relative risk (RR) and their 95 confidence intervals (95% CIs) for dichotomous outcomes and mean differences (MD) and their 95% CIs for continuous outcomes. Inverse variance random effect meta-analyses were performed when outcome data were available for at least 2 studies judged to be homogeneous about study characteristics. Between study variance τ2 was calculated with the Paule-Mandel method. Effects from meta-analyses were reported as relative risks (RR) and their 95% CIs, and heterogeneity of effects among studies was quantified with the I 2 statistic (an I 2 > 60% means high heterogeneity of effects). We primarily stratified analyses by study design (i.e. RCTs and cohort studies separately). R 3.5.1 (www.r-project.org) was used for meta-analyses.
The quality or certainty of evidence was evaluated using the GRADE methodology, which covers 5 items: risk of bias, inconsistency, indirectness, imprecision, and publication bias [17]. Quality of evidence was evaluated per specific comparison and per outcome, and described in summary of findings (SoF) tables; GRADEpro GDT was used to create SoF tables [18].
This was a systematic review of published studies, and no patients were involved in setting the research question or the outcome measures; thus, no ethics approval was required.
Results
We identified 1533 studies with our search strategy. After removing duplicates, 1103 studies were screened for eligibility by reviewing titles and abstracts. Among these, 1077 were excluded and 27 full-text articles were further assessed for eligibility. No further exclusion of full-text articles was done. Five RCTs (n = 1067) [19–23], 6 cohort studies (n = 881) [24–29], and 16 case series (n = 35,508) were included for the qualitative analysis. Then, 5 RCTs and the 6 cohort studies were included in the quantitative analysis (Supplementary Figure S1). Among RCTs, Avendaño-Solà et al. [21] and Agarwal et al. [22] included only moderate COVID-19 patients, while Li et al. [19], Gharbharan et al. [20], and Simonovich et al. [23] included patients with severe COVID-19. All RCTs and cohorts evaluated CP vs. SOC, except Simonovich et al. [23], where the control group was placebo + SOC.
Table I shows baseline characteristics of eleven studies, 5 RCTs and 6 cohorts, evaluating COVID-19 patients from Argentina, China, India, Iran, Iraq, Netherlands, Spain and the United States [19–29]. Avendaño-Solà et al. [21] included patients with SpO2 ≤ 94% but excluded those in mechanical ventilation or high-flow oxygen devices. Sample size ranged widely from 21 to 387 patients. Median age ranged between 47.8 and 73.0, severity was defined as respiratory distress, respiratory rate > 30, hypoxemia with SpO2 < 93%, PaFiO2 < 300, pulmonary infiltrates > 50%, or life-threatening disease (mechanical ventilation, septic shock, multi-organ dysfunction). Across studies, mean prevalence rates of comorbidities were: hypertension 40%, obesity 34.9%, diabetes 30.3%, and CAD 19.2%.
Table I
Baseline characteristics of included studies
Hegerova et al. [24] | Liu et al. [25] | Rasheed et al. [26] | Zeng et al. [27] | Abolghasemi et al. [28] | Salazar et al. [29] | Gharbharan et al. [20] | Li et al. [19] | Avendaño-Solá et al. [21] | Agarwal et al. [22] | Simonovich et al. [23] | ||||||||||||
---|---|---|---|---|---|---|---|---|---|---|---|---|---|---|---|---|---|---|---|---|---|---|
Design | Matched cohort | Matched cohort | Matched Cohort | Unmatched Cohort | Matched Cohort | Matched Cohort | RCT | RCT | RCT | RCT | RCT | |||||||||||
Matching variables | Age, number of comorbidities, WHO score, SOFA score and severity of illness | Intubation status and duration, length of hospital stay, O2 requirement at day 0 and administration of HCQ and AZT | Age, sex | None | Gender, age, hypertension, diabetes mellitus and CT scan score | Age, sex, BMI, diabetes, HTN, chronic pulmonary disease, CKD, hyperlipidemia, CAD and baseline ventilation requirement < 48 h of admission | None | None | None | None | None | |||||||||||
Country | United States | United States | Iraq | China | Iran | United States | Netherlands | China | Spain | India | Argentina | |||||||||||
Population | Hospitalized, severe or critically ill COVID-19 patients | Hospitalized, severe or life-threatening COVID-19 patients | Hospitalized, critically ill COVID-19 patients | Hospitalized, critically ill COVID-19 patients | Hospitalized, severe COVID-19 patients | Hospitalized, severe or life-threatening COVID-19 patients | Hospitalized, severe COVID-19 patients | Hospitalized, severe or life-threatening COVID-19 patients | Hospitalized, moderate COVID-19 patients | Hospitalized moderate COVID-19 patients | Hospitalized, severe COVID-19 patients | |||||||||||
Inclusion criteria | Severe or critical illness | Severe or life-threatening | Pneumonia with dyspnea and O2 saturation < 90% in resting state, residing in RCU | Requiring intensive care unit admission | Lung involvement by CT scan, clinical symptoms such as dyspnea, respiratory frequency ≥ 20/min, fever and cough, blood oxygen saturation (SPO2) ≤ 93% at rest on room air and ≤ 7 days since illness onset | Severe: Dyspnea, RR ≥ 30/min, O2 saturation ≤ 93% at FiO2 0.21, PaFiO2 < 300, and/or pulmonary infiltrates > 50% within 24 to 48 h (of screening). Life-threatening: respiratory failure, septic shock, and/or multiple organ failure | Mechanical ventilation and organ support OR oxygen support by mask or non-invasive ventilation | PaFiO2 ≤ 300 mmHg or SpO2 ≤ 93% or RR ≥ 30/min) or life-threatening (shock, organ failure, MV) | Pulmonary infiltrates or SpO2 ≤ 94% on room air plus within 12 days of onset of symptoms | PaFiO2 200–300 mmHg or RR > 24/min plus SpO2 ≤ 93% on room air | PaFiO2 ≤ 300 mmHg or SpO2 ≤ 93% | |||||||||||
Sample size | 40 | 195 | 49 | 21 | 189 | 387 | 86 | 103 | 81 | 464 | 333 | |||||||||||
Primary outcome | Clinical improvement at days 7 and 14 | O2 supplementation improvement and survival rate at days 1, 7, and 14 | Safety of CP within 3 h, time to PCR conversion, survival or death rate | Death or recovery (discharge) | Survival and hospital length of stay | Mortality within 28 days post day 0 | Mortality rate at 15 days | Clinical improvement within 28 days | Progression of patients to categories 5–7 at day 15 | Progression to severe disease (PaO2/FiO2 < 100) within 28 days or all-cause mortality at 28 days | Clinical status at 30 days measured with a 6 point ordinal scale | |||||||||||
Intervention | 1 unit of ABO compatible CP | 2 units of 250 ml CP | Highly elevated SARS-CoV-2 IgG CP (unspecified volume) | 300 ml of CP | 500 ml of CP over 4 h | 1 unit of plasma, second dose if needed | 300 ml of CP | 4–13 ml/kg ABO-compatible, 10 ml for 15 min followed by 100 ml/h | 1 unit 250–300 ml of CP | 2 units 200 ml of CP | 5–10 ml/kg (between 400–600 ml), IgG Ab titer of 1 : 400 or higher | |||||||||||
Comparator | Additional therapies, other than CP | AZT and HCQ, but no CP | Standard of care | No CP | No CP | Standard of care | Standard of care | Standard treatment | Standard of care | Standard of care | Placebo and standard of care | |||||||||||
Follow-up time | 14 days | 14 days | 5 days | Intervention group = 45.4 days (37.8–59) | Unspecified | 28 days | 15 days | 28 days | 15 days | 28 days | 30 days | |||||||||||
Control group = 31 days (30–36) | ||||||||||||||||||||||
Concomitant treatment | AZT, HCQ | AZT, HCQ | Unspecified | ATB, antivirals, traditional Chinese medicine, IVIG, glucocorticoids, RRT | HCQ, L/R, anti-inflammatories | Steroids, AZT, HCQ, L/R, remdesivir, ribavirin, TCZ | AZT, CQ, L/R, TCZ, anakinra | Antivirals, steroids, antibacterial, antifungal, IVIG, IFN | Steroids, AZT, HCQ, L/R, remdesivir, heparin, TCZ | Antivirals, HCQ, remdesivir, L/R, oseltamivir, antibiotics, steroids, TCZ, oxygen | Steroids, HCQ, L/R, TCZ, oxygen, ivermectin | |||||||||||
CP | C | CP | C | CP | C | CP | C | CP | C | CP | C | CP | C | CP | C | CP | C | CP | C | CP | C | |
(n = 20) | (n = 20) | (n = 39) | (n = 156) | (n = 21) | (n = 28) | (n = 6) | (n = 15) | (n = 115) | (n = 74) | (n = 136) | (n = 251) | (n = 43) | (n = 43) | (n = 52) | (n = 51) | (n = 38) | (n = 43) | (n = 235) | (n = 229) | (n = 228) | (n = 105) | |
Age, mean (SD) or median (IQR) | 60 (29–95) | NA | 55 (13) | NA | 55.66 (17.83) | 47.82 (15.36) | 61.5 (31.5–77.8) | 73 (60–79) | 54.41 (13.71) | 56.83 (14.98) | NA | NA | 62 (10.37) | 64.5 (16.3) | 70 (62–80) | 69 (63–76) | 60.5 (46–74) | 58 (51-73) | 52 (42–60) | 52 (41–60) | 62.5 (53.72.5) | 62 (49–71) |
Male, n (%) | NA | NA | 25 (64) | NA | 12 (57.1) | NA | 5 (83.3) | 11 (73.3) | 67 (58.3) | 37 (50) | 84 (61.8) | 145 (59.2) | 29 (67) | 33 (77) | 27 (51.9) | 33 (64.7) | 20 (52.6) | 24 (55.8) | 177 (75.3) | 177 (77.3) | 161 (70.6) | 64 (61.0) |
Comorbidities, n (%): | ||||||||||||||||||||||
HTN | 12 (60) | NA | NA | NA | NA | NA | 1 (16.7) | 3 (20) | 22 (27.5) | 19 (38) | 62 (45.6) | 124 (49.4) | 11 (26) | 11 (26) | 29 (55.8) | 27 (59.2) | 20 (52.6) | 12 (47.9) | 92 (39.1) | 81 (35.5) | 111 (48.7) | 48 (45.7) |
DM | 9 (45) | NA | 8 (21) | NA | NA | NA | 1 (16.7) | 5 (33.3) | 27 (33.8) | 16 (32) | 52 (38.2) | 114 (45.4) | 13 (30) | 8 (19) | 9 (17.3) | 12 (23.5) | 12 (31.6) | 5 (11.6) | 113 (48.1) | 87 (38.2) | 40 (17.5) | 21 (20) |
Dyslipidemia | NA | NA | NA | NA | NA | NA | NA | NA | NA | NA | 38 (27.9) | 88 (35.1) | NA | NA | NA | NA | NA | NA | NA | NA | NA | NA |
Asthma | NA | NA | 3 (8) | NA | NA | NA | NA | NA | NA | NA | NA | NA | NA | NA | NA | NA | NA | NA | NA | NA | 9 (3.9) | 5 (4.8) |
Obesity | 4 (20) | NA | 21 (54) | NA | NA | NA | NA | NA | NA | NA | 84 (61.8) | 149 (59.4) | NA | NA | NA | NA | NA | NA | 16 (6.8) | 17 (7.4) | 104 (45.6) | 52 (49.5) |
CAD | NA | NA | NA | NA | NA | NA | 1 (16.7) | 0 (0) | NA | NA | 9 (6.6) | 28 (11.2) | 9 (21) | 11 (26) | 25 (48.1) | 19 (31.2) | 6 (15.8) | 9 (20.9) | 15 (6.4) | 17 (7.4) | 8 (3.5) | 3 (2.9) |
CKD | NA | NA | 3 (8) | NA | NA | NA | 0 (0) | 1 (6.7) | NA | NA | 13 (9.6) | 38 (15.1) | 5 (12) | 6 (14) | 2 (3.9) | 4 (7.8) | 2 (5.3) | 2 (4.7) | 8 (3.4) | 9 (3.9) | 10 (4.4) | 4 (3.8) |
Liver Disease | NA | NA | NA | NA | NA | NA | 0 (0) | 2 (13.3) | NA | NA | NA | NA | 0 (0) | 1 (2) | 5 (9.6) | 5 (9.8) | NA | NA | 0 | 2 (0.9) | NA | NA |
[i] RCT – randomized controlled trial, HTN – hypertension, DM – diabetes mellitus, CVD – coronary artery disease, CKD – chronic kidney disease, HCQ – hydroxychloroquine, CQ – chloroquine, AZ – azithromycin, CP – convalescent plasma, TCZ – tocilizumab, C – control, NA – not applicable, ATB – antibiotics, IVIG – intravenous immunoglobulin, RRT – renal replacement therapy, IFN – interferon, LOS – length of hospital stay, L/R – lopinavir/ritonavir.
There was heterogeneity of the timing of CP administration from symptom appearance or COVID-19 diagnosis to randomization, ranging from 8 days [21, 23] to 41 days [22] (Supplementary Table SI). Antibody titers of donors were also heterogeneous, ranging from at least > 1 : 20 [22] to at least > 1 : 640 [19]. Also, the percentage of positivity of antibodies in patients at randomization ranged from 0% [19] to 83% [22].
Outcomes such as all-cause mortality, clinical improvement, and serious and overall adverse events are shown in Supplementary Table SII. All-cause mortality was the common outcome in every study, clinical improvement was only reported in 3 RCTs and 2 cohorts, clinical worsening or progression in 2 RCTs, and need for invasive ventilation in 2 RCTs. Adverse events were found in 3 RCTs and 3 cohorts, and SAEs in 3 RCTs. Details of included RCTs and cohorts are described in the supplementary material (Supplementary Appendix S2).
Our study also included 16 case series [12, S1–S15] with 35 508 patients from all over the world. Most patients were male and over 51 years old. Dosing and frequency were very heterogeneous, ranging from 200 ml to 500 ml, with 200 ml being the most used dosage. Almost all patients received 1 dose; however, some of them were scheduled to receive 2 or even 3 doses. The most repeated primary outcomes were mortality and clinical improvement. Other studies evaluated clinical scores such as SOFA and PaFiO2 as well as adverse events.
Quality of evidence was low or very low for most of clinical and composite safety outcomes (Table II and Supplementary Table SIII). Gharbharan et al. [20] and Simonovich et al. RCTs [23] showed some concerns of bias in the randomization process, the Li et al. [16] RCT showed some concerns of bias in the randomization process and in deviation from intended interventions, the Avendaño-Solà et al. [18] RCT showed high risk of bias in the randomization process, and Agarwal et al. [19] reported low risk of bias (Supplementary Figure S2). The 6 cohort studies showed serious risk of bias due to potential confounding, and selection of participants for the study; 2 cohorts had serious risk of bias in classification of interventions and 3 cohorts had serious risk of bias in selection of the reporting results (Supplementary Figure S3).
Table II
Summary of findings table of convalescent plasma compared to standard of care in hospitalized COVID-19 patients
Outcomes | Anticipated absolute effects* (95% CI) | Relative effect (95% CI) | No of participants (studies) | Certainty of the evidence (GRADE) | |
---|---|---|---|---|---|
Risk with standard of care | Risk with convalescent plasma | ||||
All-cause mortality in severe patients follow-up: range 15 days to 28 days | 24 per 100 | 15 per 100 (8 to 27) | RR 0.60 (0.33 to 1.10) | 189 (2 RCTs) | ⨁⨁◯◯ LOWa,b |
All-cause mortality in moderate patients follow-up: range 15 days to 28 days | 13 per 100 | 8 per 100 (1 to 50) | RR 0.60 (0.09 to 3.86) | 545 (2 RCTs) | ⨁◯◯◯ VERY LOWc,d,e |
All-cause mortality in severe patients follow-up: range 5 days to 45 days | 19 per 100 | 12 per 100 (9 to 17) | RR 0.66 (0.49 to 0.91) | 881 (6 observational studies) | ⨁◯◯◯ VERY LOWf,g |
Clinical improvement in severe patients assessed with: patient discharged alive or improvement of 2 categories on a 6-point severity scale (from 1 (discharge) to 6 (death)) follow-up: mean 28 days | 43 per 100 | 55 per 100 (36 to 75) | HR 1.40 (0.79 to 2.49) | 103 (1 RCT) | ⨁⨁◯◯ LOWh,i |
Improvement in severe patients assessed with: undefined using adjusted proportional odds models of WHO 8-point ordinal scale follow-up: mean 15 days | 14 per 100 | 17 per 100 (8 to 35) | OR 1.30 (0.52 to 3.32) | 86 (1 RCT) | ⨁◯◯◯ VERY LOWj,k,l |
Clinical worsening in moderate patients assessed with: progression to categories 5, 6, and 7 on a 7-point ordinal scale (from 1 (discharge) to 7 (death)) follow-up: mean 15 days | 14 per 100 | 0 per 100 (0 to 0) | not estimable | 81 (1 RCT) | ⨁◯◯◯ VERY LOWc,m |
Progression to severe disease in moderate patients assessed with: PaO2/FiO2<100 any time within 28 days follow-up: mean 28 days | 7 per 100 | 7 per 100 (4 to 14) | RR 0.97 (0.51 to 1.86) | 464 (1 RCT) | ⨁⨁⨁◯ MODERATEn |
Need of invasive ventilation in moderate patients follow-up: mean 28 days | 8 per 100 | 7 per 100 (4 to 12) | RR 0.85 (0.47 to 1.55) | 545 (2 RCTs) | ⨁◯◯◯ VERY LOWc,o |
Adverse events in severe patients follow-up: mean 28 days | 0 per 100 | 0 per 100 (0 to 0) | not estimable | 103 (1 RCT) | ⨁◯◯◯ VERY LOWh,p |
Adverse events in moderate patients follow-up: mean 28 days | 3 per 100 | 3 per 100 (1 to 8) | RR 0.97 (0.32 to 2.98) | 464 (1 RCT) | ⨁⨁⨁◯ MODERATEq |
Severe adverse events in severe patients assessed with: pulmonary edema, severe allergic reaction, anaphylactic shock follow-up: mean 28 days | 0 per 100 | 0 per 100 (0 to 0) | RR 1.92 (0.16 to 22.69) | 189 (2 RCTs) | ⨁◯◯◯ VERY LOWa,r |
Severe adverse events in moderate patients assessed with: pulmonary edema, severe allergic reaction, anaphylactic shock follow-up: mean 15 days | 16 per 100 | 16 per 100 (6 to 43) | RR 0.97 (0.36 to 2.64) | 81 (1 RCT) | ⨁◯◯◯ VERY LOWc,s |
a RoB 2.0: Gharbharan et al. RCT had some concerns of risk of bias in the randomization process; Li et al. had some concerns of risk of bias in the randomization process and in deviation from the intended interventions.
c RoB 2.0: Avendaño-Solà et al. RCT had high risk of bias in the randomization process; Agarwal et al. RCT had low risk of bias.
f RoB ROBINS-I: All 6 cohorts had serious risk of bias due to residual confounding and selection of participants for the study.
h RoB 2.0: Li et al. had some concerns of risk of bias in the randomization process and in deviation from the intended interventions.
k Indirectness: Improvement of the WHO 8-point ordinal scale was not defined in manuscript or supplement of Gharbharan et al. RCT.
Convalescent plasma had no effect on the risk of all-cause mortality vs. SOC in 2 RCTs [16, 17] of severe COVID-19 patients (RR = 0.60, 95% CI: 0.33–1.10) (Figure 1). Convalescent plasma did not have an effect on all-cause mortality vs. SOC in 2 RCTs [18, 19] of moderate COVID-19 patients (RR = 0.60, 95% CI: 0.09–3.86) (Figure 2). Among cohort studies at serious risk of bias [20–25], the reduction of all-cause mortality was significant vs. SOC (RR = 0.66, 95% CI: 0.49–0.91) (Figure 3). Clinical improvement or improvement was scarcely and heterogeneously reported for the comparison of CP vs. SOC. Convalescent plasma did not have an effect on the need for invasive ventilation vs. SOC in 2 RCTs [18, 19] of moderate COVID-19 patients (RR = 0.85, 95% CI: 0.47–1.55) (Supplementary Figure S4). Adverse events and SAEs were very scarce and similar between CP and SOC; SAEs between CP and SOC were similar in 2 RCTs [16, 17] of severe COVID-19 patients (RR = 1.92, 95% CI: 0.16–22.69). There was not enough information about secondary outcomes to perform a meta-analysis.
Figure 3
Effect of convalescent plasma on all-cause mortality in cohort studies in severe COVID-19 patients
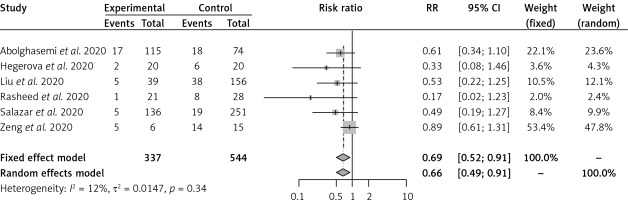
Convalescent plasma had no effect on the risk of all-cause mortality vs. control (placebo plus SOC) in 3 RCTs [19, 20, 23] of severe COVID-19 patients (RR = 0.75, 95% CI: 0.48–1.16) (Supplementary Figure S5). Clinical improvement was not different between CP and control (placebo plus SOC) in 2 RCTs [19, 23] of severe COVID-19 patients (HR = 1.07, 95% CI: 0.82–1.40) (Supplementary Figure S6). In comparison to the control (placebo plus SOC), CP had no effect on adverse events (RR = 1.07, 95% CI: 0.90–1.28) [19, 23] or SAEs (RR = 1.33, 95% CI: 0.84–2.10) [19, 20, 23] in severe COVID-19 patients (Supplementary Figures S7 and S8). Although subgroup findings were restricted by the limited number of outcomes and RCTs, there were no differences of CP effects across RCTs by severity of disease, by type of control, or by other differences across RCTs (timing of CP administration, by titers of antibodies in donors, and by percentage of positivity of antibodies to SARS-CoV-2 at randomization) (all p for interaction > 0.2).
We identified 56 ongoing RCTs taking place worldwide; 36 studies compare CP to standard treatment of care while the other 20 use a placebo such as standard plasma, albumin, immunoglobulin, saline or Ringer’s lactate as the comparator. Almost all of them include serious or critically ill patients. Supplementary Table SIV describes their details.
Discussion
Convalescent plasma did not show an all-cause mortality benefit compared to placebo or standard of care in RCTs of severe and moderate hospitalized COVID-19 patients. However, we found a significant all-cause mortality benefit among 6 cohorts at serious risk of bias, 5 of them with some degree of matching for potential confounders. There was a scarcity of outcome data about clinical improvement or worsening, need of invasive ventilation, or length of stay across studies. Most of the studies reported scarce or no adverse events or serious adverse events, and there were no differences between CP and standard of care or placebo plus standard of care arms. Three out of 5 RCTs were stopped prematurely due to the lack of new patients or the presence of neutralizing antibodies at baseline. Quality of evidence was low or very low for most clinical and composite safety outcomes.
There are previously published reviews [30–34] of the efficacy and harms of the use of CP in severely ill patients with COVID-19: 2 narrative reviews [30, 34], and 3 systematic reviews (SR) [31–33]. However, none of these reviews evaluated all the studies we included and only Joyner et al. [30] included a meta-analysis. Joyner et al. [30] evaluated 3 RCTs, 5 matched-control studies, and 4 case series and concluded that mortality was diminished in the CP group among the RCTs (OR = 0.46) and among the matched-control studies (OR = 0.41). Nevertheless, the authors did not report 95% CI, mistakenly used OR and a fixed effects model for the meta-analysis, and misclassified the Rasheed et al. study [26] as an RCT when it is a matched-control cohort study.
Valk et al. [32] performed an SR that included only 1 RCT and 3 controlled non-randomized studies of interventions (NRSI) in the quantitative analysis. They reported uncertainty for the effect of CP on all-cause mortality (RR = 0.89, 95% CI: 0.61–1.31), on clinical improvement at 7 days (RR = 0.98, 95% CI: 0.30–3.19), at 14 days (RR = 1.85, 95% CI: 0.91–3.77), and at 28 days (RR = 1.20, 95% CI: 0.80–1.81). They did not perform a meta-analysis due to critical risk of bias in the controlled NRSIs. Valk et al. [32] performed a narrative review that included 8 case series, 1 single-arm intervention study, and no RCTs. Results of this study showed inconsistent data due to their high risk of bias and a low reporting quality. All their studied patients were alive at the end of the reporting period but not all had been discharged.
Rajendran et al. [33] was an SR that included 5 studies (1 pilot study, 1 preliminary communication, 1 novel report, 1 report case and 1 descriptive study) from China and South Korea. The heterogeneity of designs did not allow them to perform a meta-analysis. These authors reported that CP use in variable doses may have a beneficial effect on mortality rate among COVID-19 patients, but they stated that findings of zero mortality in those studies could also be due to a synergistic effect of the multiple other agents received by those patients. They also reported that CP may cause an improvement in clinical status, and with very minimal or no adverse events.
Finally, a pre-print of an SR by Pimenoff et al. [34] included ten studies with only 61 patients and neither specification of each type of study nor a PRISMA figure. There were no significant differences in time to clinical improvement between genders, between those with or without comorbidities and between those receiving CP within the first week of symptoms or after 3 weeks of symptoms onset. The authors did not analyze mortality or adverse events.
There were 2 previous SRs which evaluated CP in non-COVID-19 patients. Sun et al. [35] evaluated 40 studies of CP in infectious diseases such as SARS, MERS, pandemic influenza and Ebola, finding a significantly lower mortality rate in the intervention group (OR = 0.32, 95% CI: 0.19–0.52). Devasenapathy et al. [36] pooled 4 RCTs on influenza patients and reported no effect of CP on mortality (RR = 0.94, 95% CI: 0.49–1.81), clinical improvement (OR = 1.04, 95% CI: 0.69–1.64), and length of hospital stay (MD = –1.62, 95% CI: –3.82 to –0.58). Furthermore, they did not find an association between CP and serious adverse events (RR = 0.85, 95% CI: 0.56–1.29).
Although it is reasonable to think that early administration of CP may improve mortality in COVID-19, this has been found in a case series study by Cheng et al. [8] comparing < 14 days vs. > 14 days of COVID-19 (mortality rates: 6.3% vs. 21.9%, respectively). Also, the FDA emergency use authorization of CP was based on subgroup analyses by time of administration (7-day mortality rate was 8.7% (95% CI: 8.3–9.2%) in patients transfused within 3 days of COVID-19 diagnosis but 11.9% (11.4–12.2%) in patients transfused 4 or more days after diagnosis (p < 0.001)), and subgroup analyses by IgG concentration of CP (7-day mortality rate was 8.9% with high IgG levels vs. 13.7% with low IgG levels) in another large case series by Joyner et al. [12]. Case series studies are exploratory and cannot provide estimates of efficacy or effectiveness as there is no control group; findings in case series studies may be due to the effect of confounders or other differences in the groups being compared.
The Agarwal et al. RCT [22] was different from other RCTs. In this study, the median time from COVID-19 diagnosis to intervention was 41 days, which is longer than the 8 to 30 day range from COVID-19 symptoms to randomization of the other 4 RCTs [19–21, 23]. In addition, Agarwal et al. reported that 83% of the intervention and control individuals had positive total neutralizing SARS-CoV-2 antibodies at randomization, and that only 64% of donors reached antibodies titers of at least 1 : 20 (median titer of 1 : 40). These findings differed from the other 4 RCTs, where the percentage positivity of neutralizing SARS-CoV-2 antibodies ranged between 0% [19] and 80% [20], and the antibody titers of donors ranged between at least 1 : 80 [20, 21] and at least 1 : 640 [19]. However, there were no differences of CP effects across these RCTs.
Our study has several strengths. First, we performed a systematic review and an extensive search in 5 engines, 1 pre-print website, and 3 clinical trial registries. Second, our study provides the most up-to-date and recent data until November 24, 2020 compared to the other SRs. Third, we have assessed risk of bias for RCTs and cohorts using the latest tools by Cochrane, RoB 2.0. and ROBINS-I, respectively. Fourth, we also evaluated the quality or certainty of evidence per outcome among all evaluated controlled studies using GRADE methodology. Fifth, in addition to the SR, we could meta-analyze all-cause mortality in 2 similar RCTs of severe and 2 RCTs of moderate COVID-19 patients; cohorts were meta-analyzed independently. Sixth, we formally evaluated adverse event reporting and test differences between CP and controls groups. Finally, we ran an extensive search of the ongoing parallel RCTs evaluating efficacy and safety of CP in COVID-19 patients worldwide.
In conclusion, in PCR-confirmed, COVID-19 hospitalized adult patients, there is a lack of fully powered and adequately reported RCTs evaluating the efficacy and safety of CP. One RCT was stopped early due to lack of new patients in Wuhan, China, a second RCT was stopped prematurely due to presence of neutralizing antibodies at baseline, had scarce data about clinical improvement and did not report adverse events, a third RCT evaluated moderately ill patients, had high risk of bias, and was stopped early due to lack of new patients, a fourth RCT assessed moderately ill patients and had low risk of bias, and a fifth RCT evaluated CP vs. placebo plus SOC in severe patients and had some concerns of risk of bias. These trials did not show all-cause mortality benefit with the use of CP vs. SOC or placebo plus SOC in both moderate and severe COVID-19 patients. Although the cohort studies showed a statistically significant effect on all-cause mortality, we identified overall serious risk of bias in all of them. Individual RCTs showed no effect of CP vs. SOC on clinical improvement or worsening or in need of mechanical ventilation, and no effect of CP vs. placebo plus SOC on clinical improvement. There were no differences in adverse events or serious adverse events between CP and SOC or placebo plus SOC, and these events were scarce across studies.
Overall, the identified studies showed heterogeneous data about timing of administration of CP, antibody titers in donors, and positivity of antibodies at baseline in randomized patients. Controlled studies have shown no effects and low or very low quality of evidence for clinical and adverse effects on these patients, and therefore CP should not be recommended for the treatment of hospitalized COVID-19 patients. Ongoing randomized controlled trials will provide more information on the effects of CP on clinical and safety outcomes in the near future.