Introduction
MicroRNAs (miRNAs) are basically 23 nucleotide-long RNA molecules that control the expression of 30% of human genes by binding to the mRNAs and enforcing their post-transcriptional repression [1]. The miRNAs are involved in different cellular and physiological processes such as the cell cycle and apoptosis [2]. Accumulating evidence suggests that several microRNAs are aberrantly expressed in patients with diabetes mellitus, neurological disorders and malignancies. Therefore, miRs are considered to be prospective therapeutic targets for the management of these diseases [3]. It has been shown that miR-125b is aberrantly expressed in polycystic ovary syndrome (PCOS) [4]. However, its role in the pathogenesis of PCOS has yet not been investigated. Being one of the common types of endocrine disorders, PCOS affects approximately 4–10% of women at reproductive age [5, 6]. This syndrome generally refers to hyper-androgenism, insensitivity to insulin and oligoovulation [7]. Previous investigations performed over the last few decades have confirmed that PCOS is often linked with an increased risk of type 2 diabetes mellitus and the PCOS patients with mild hyperandrogenism but normal ovulation have the risk of developing this chronic disorder in future [8, 9]. Additionally, currently employed treatment strategies for PCOS include insulin sensitizers such as metformin, but these drugs can cause adverse effects that negatively affect the quality of life of patients [10–12]. This study aims to explore the role of miR-125b in PCOS and the therapeutic potential of miR-125b for the management of affected patients
Material and methods
Clinical samples and cell lines
The patients were recruited at the Department of Gynecology, The First Affiliated Hospital of Chengdu Medical College, China, from 2016 to 2018. The study was approved by the research ethics committee under approval number AHC/C270 of 2018 and informed consent was obtained a priori from all the women. All the women were in the same phase of the menstrual cycle and the mean age for normal and PCOS women was 34.5 and 34 respectively. We recruited 12 women with PCOS, undergoing laparoscopic investigation for infertility or ovarian drilling for ovulation induction. The diagnosis of PCOS was established according to the revised Rotterdam European Society of Human Reproduction and Embryology/American Society for Reproductive Medicine criteria (2003) [13]. The PCOS subjects should present at least two of the following criteria: oligo- and/or anovulation, clinical hyperandrogenism and/or biochemical hyperandrogenism and polycystic ovaries after exclusion of other etiologies (e.g. congenital adrenal hyperplasia, androgen-secreting tumors, and Cushing’s syndrome). In the control group, we recruited 12 normally menstruating women who were undergoing laparoscopic sterilization, hysterectomy for benign conditions, and diagnostic laparoscopy for pelvic pain. The ovarian granulosa KGN cell line and the normal ovarian cell line SV40 were obtained from Cell Bank of Chinese Academy of Science (Shanghai, China) and maintained as described previously [14]. The KGN cell line has been used as an in vitro model for the study of PCOS as mentioned previously [14].
cDNA synthesis, qRT-PCR and transfections
Firstly the RNA of the cell lines and the tissue samples was extracted with the help of TRIzol reagent and then cDNA was generated from the isolated RNA using cDNA synthesis kit (Invitrogen). SYBR Green master mix was then used to quantify the relative expression of the miR-125b by the 2–ΔΔCq method. Lipofectamine 2000 reagent was employed to carry out the transfections of the miR-125b mimics (5´AGUGUUCAAUCCCAGAGUCCC 3´) and NC (5´ GUAGGAGUAGUGAAAGGCC 3´) following the manufacturer’s guidelines. The primers for miR-125b were forward 5′-GCCCTCCCTGAGACCTCAA-3′; and reverse 5′-GTGCAGGGTCCGAGGT-3′ and for cyclin B1 forward 5’-AAAGGCGTAACTCGAATGGA3´ and reverse 5’-CCGACCTTTTATTGAAGAGCA-3´.
Cell counting kit-8 (CCK-8) assay
After 48 h of transfection, KGN cells were grown in 96-well plates in three replicates at the density of 1.0 × 103 cells/well. The proliferation rate and the viability of the KGN cells were estimated by Cell Counting Kit-8 following the guidelines of the manufacturer. Around 10 µl of CCK-8 reagent was added into the wells at specific time intervals (12, 24, 48, 72 and 96 h) and the plates were then subjected to incubation for about 2 h at 37°C. Viability of the KGN cells was then measured by taking the absorbance at 450 nm.
Target identification
The miR-125b targets were identified with the help of TargetScan version 7.2 online software (http://www.targetscan.org).
Dual-luciferase reporter assay
The miR-125b mimics or NC were co-transfected with plasmid pGL3-cyclin B1´-UTR-WT or pGL3-cyclin B1´-UTR-MUT into KGN cells. Dual-luciferase reporter assay (Promega) was carried out at 48 h after transfection. Renilla luciferase was used for normalization.
DAPI staining for apoptosis
For DAPI staining, the transfected ovarian granulosa KGN cells (0.6 × 106) were grown in 6-well plates. After an incubation period of around 12 h, the KGN cells were subjected to incubation for 24 h at 37°C. As the cells sloughed off, 25 µl cell cultures were put onto glass slides and subjected to staining with DAPI. The slides were covered with a cover slip and examined with a fluorescent microscope.
Cell cycle analysis
After transfection the KGN cells were subjected to culturing for 24 h at 37°C. The KGN cells were harvested and the PBS washed. Afterwards, the KGN cells were stained with propidium iodide (PI) and the distribution of the cells in cell cycle phases was assessed by a FACS flow cytometer.
Western blotting
KGN cells were firstly subjected to washing with ice-cold PBS and then suspended in a lysis buffer at 4°C and then shifted to 95°C. Thereafter, the protein content of each cell extract was determined by Bradford assay. About 40 µg of protein was loaded from all samples and separated by SDS-PAGE before being shifted to polyvinylidene fluoride membrane. The membranes were then subjected to treatment with TBS and then exposed to primary antibodies at 4°C. Thereafter, the cells were administered with appropriate secondary antibodies and the proteins of interest were visualized by enhanced chemiluminescence reagent.
Results
miR-125b is upregulated in PCOS ovaries and KGN ovarian granulosa cells
The transcript profile of miR-125b was examined in normal and polycystic ovary specimens by qRT-PCR (Figure 1 A). The results showed that miR-125b exhibited aberrant expression and was significantly upregulated (p < 0.05) in all polycystic ovary tissues (Figure 1 B). The expression of miR-125b was almost twice as high in the ovarian tissues of PCOS patients when compared to the ovarian tissues of healthy women. The miR-125b levels were also examined in KGN PCOS cells and normal SV40 ovarian cells. It was found that miR-125b expression was around 5.6-fold higher in the KGN cells in comparison to the normal SV40 cells (Figure 1 B).
Inhibition of miR-125b KGN cells triggers G2/M arrest
Next, we sought to decipher the role of miR-125b in PCOS and for that the KGN cells were subjected to transfection with either the miR-125b inhibitor or NC. The suppression of miR-125b in KGN cells was confirmed by qRT-PCR, which showed that transfection of the KGN cells with miR-125b inhibition caused around 10-fold reduction in miR-125b expression relative to NC transfected cells (Figure 2 A). Next the proliferation rate of the NC and miR-125b inhibitor transfected KGN cells was estimated by CCK-8 assay and it was revealed that the transfection of miR-125b inhibitor in the KGN cells caused a significant decline in their proliferation rate (Figure 2 B). Next, distribution of NC and miR-125b inhibitor KGN cells was estimated by flow cytometry to reveal the underlying reason for reduction in the proliferation of miR-143 mimic transfected cells. The flow cytometry analysis showed that miR-125b inhibitor transfection (i.e., miR-125b suppression) caused G2/M arrest of the KGN cells (Figure 2 C). The percentage of the G2/M KGN cells increased from 13.5% in the control to 55.1% in the miR-125b inhibitor transfected KGN cells. Next DAPI staining was also performed to determine whether miR-125b inhibition triggers apoptosis in the KGN cells. DAPI staining together with caspase 3 expression analysis revealed that miR-125B did not induce apoptotic cell death in the KGN cells transfected with NC or miR-125b inhibitor (Figures 2 D and E).
Figure 2
miR-125 inhibition suppresses KGN cell proliferation. A – Expression of miR-125b in negative control (NC) or miR-125 inhibitor (miR-125 Inh) transfected KGN cells. B – Cell viability of negative (NC) or miR-125 Inh transfected KGN cells. C – Cell cycle analysis of KGN cells transfected with NC or miR-125 Inh. D, E – DAPI staining of KGN cells transfected with NC or miR-125 Inh. The experiments were performed in triplicate (*p < 0.05)
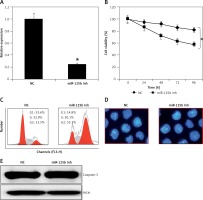
miR-125b targets cyclin B1 in gastric cancer cells
The target of miR-125b in KGN ovarian granulosa cells was determined by online TargetScan analysis. Cyclin B1 was identified as the potential target of miR-125b in KGN cells (Figure 3 A), which was further confirmed by the luciferase assay (Figure 3 B) and therefore the expression levels of cyclin B1 were investigated in NC as well as the miR-125b inhibitor transfected KGN ovarian granulosa cells. The results indicated that expression of cyclin B1 was considerably upregulated as depicted by the western blot analysis (Figure 3 C).
Figure 3
miR-125b exerts its effects by targeting cyclin B1. A – Identification of cyclin B1 as the target of miR-125b. B – Dual luciferase assay. C – Western blots showing expression of cyclin B1 in NC or miR-125b Inh transfected KGN cells. D – qRT-PCR expression of cyclin B1 in NC or Si-cyclin B1 transfected KGN cells. E – Western blots showing the expression of cyclin B1 in NC or Si-cyclin B1 transfected KGN cells. F – Cell viability of the NC or Si-cyclin B1 transfected cells. The experiments were performed in triplicate (*p < 0.05)
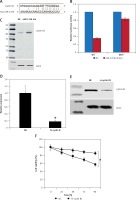
Next, we sought to reveal the impact of the cyclin B1 silencing on the viability of KGN cells. Therefore, the KGN cells were transfected with NC or Si-cyclin B1 and cyclin-B1 silencing was confirmed by qRT-PCR analysis, which showed 5.7-fold depletion of the cyclin B1 expression in Si-cyclin B1 transfected cells relative to the NC transfected KGN cells (Figure 3 D). The silencing of the cyclin B1 was also confirmed by western blot analysis (Figure 3 E). Further, the results of the CCK-8 showed that Si-cyclin B1 transfection (i.e., cyclin B1 silencing) caused significant reduction in KGN cell viability (Figure 3 F). Cell cycle analysis of the NC or Si-cyclin B1 cells showed that miR-125b inhibition triggers G2/M arrest (Figure 4 A). The percentage of the G2/M phase KGN cells increased from 20.4% in NC transfected KGN cell to 61% in Si-cyclin B1 transfected cells. However, miR-125b silencing in the KGN cells did not trigger apoptotic cell death as indicated by DAPI and caspase-3 expression (Figures 4 B and C).
Figure 4
Silencing of cyclin B1 triggers G2/M phase arrest. A – Flow cytometric analysis showing the cell cycle distribution of KGN cells transfected with NC or Si-cyclin B1. B – DAPI staining of the NC or Si-cyclin B1 transfected KGN cells. C – Expression of caspase-3 in NC or Si-cyclin B1 treated KGN cells. The experiments were performed in triplicate (*p < 0.05)
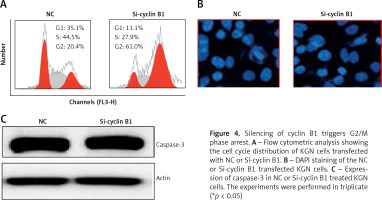
Cyclin B1 is essential for miR-125b mediated effects on KGN cell proliferation
Since miR-125b inhibition and cyclin B1 silencing both inhibited the growth of the KGN cells, we sought to find out if miR-125b overexpression could nullify the impact of cyclin B1 silencing in KGN cells. Interestingly, it was observed that miR-125b overexpression in the Si-cyclin B1 transfected KGN had no impact on the cyclin B silencing induced effects on the KGN proliferation (Figure 5 A). Nonetheless, overexpression of cyclin B1 in the miR-125b inhibitor transfected KGN cells rescued the effects of miR-125b inhibition on the viability, indicating that cyclin B1 is imperative for miR-125b mediated effects on the KGN proliferation (Figure 5 B).
Discussion
The PCOS is one of the most commonly diagnosed metabolic and endocrine abnormalities [5]. Although PCOS affects up to 10% of the women at reproductive age, the underlying reason for its pathogenesis has not been clarified yet. The pathogenesis of PCOS has been characterized by the alteration of gonadotropin production, upsurge of steroidogenic enzymes, and insensitivity to insulin and oligoovulation, which impairs fertility [6]. Currently insulin sensitizers such as metformin are used for the treatment of PCOS, but these drugs are associated with adverse effects. Therefore, there is a need to develop more efficient and safer treatment strategies for the management of PCOS. Over the years, miRs have been described as potential therapeutic targets for the management of diseases [3]. The possible modes of action for miRNA within the pathophysiology of PCOS have only been sparsely investigated, and thus there are only a few studies that investigate the relationship between miRNA and PCOS. It is believed that miRs may act as potential targets for the pharmacological agents in PCOS patients. For example, it has been shown that miR-93 controls the proliferation of KGN cells [13] and there is overexpression of miR-125b [4] in polycystic ovaries. Moreover, it has been shown that miR-93 is overexpressed and this overexpression inhibits GLUT4 expression in adipose tissues of PCOS patients [15]. The findings of the present study indicate that miR-125b is considerably upregulated in the polycystic ovaries as well the ovarian granulosa KGN cell lines. When the expression of miR-125b is suppressed, the proliferation of KGN cells is inhibited by inducing G2/M cell cycle arrest. These results comply with previous investigations where miRs have been reported to be aberrantly expressed in polycystic ovaries. For instance, miR-21, miR-27b, miR-103 and miR-155 tend to show enhanced expression in PCOS patients [16]. Another study reported that miR-21 and 146a expression was enhanced in PCOS patients and this overexpression was associated with DNA damage in a mouse model of PCOS [17]. Additionally, it was demonstrated that miR-125b exerts its inhibitory effects by modulating cyclin B1 expression. Cyclin B1 silencing resulted in similar effects on the viability of KGN cells as that of miR-125b silencing. Cyclin B1 has been found to control the transition of the cells from G2 phase to M phase during the cell cycle [18]. This further supports our results that miR-125b suppresses the proliferation of granulosa cells by targeting cyclin B1 and cyclin B1 overexpression could overcome the effects of miR-125b suppression on the proliferation of KGN cells.
In conclusion, it can be concluded that miR-125b regulates the viability of the ovarian granulosa cells in PCOS patients, and thus miR-125 might be addressed as a potential therapeutic target for the management of PCOS. Further research should be performed to clarify the role of miR-125 in PCOS pathogenesis.