Introduction
Atrial fibrillation (AF) is one of the most common arrhythmias in cardiovascular diseases. When AR occurs, the blood in the atrium cannot be pumped out in a normal and orderly manner, resulting in haemodynamic disorder, which increases the risk of thrombosis and thromboembolism [1, 2]. The heart mainly generates adenosine triphosphate (ATP) through mitochondrial oxidation of fatty acid (FA, 60–90%), glucose, and lactic acid (10–40%) to maintain the energy required for myocardial contraction-diastole [3]. Myocardium can use a variety of substrates to produce energy, the most important of which are fatty acids and glucose, followed by lactic acid, ketone bodies, amino acids, etc. Murashige et al. [4] conducted a comprehensive quantitative description of the metabolism of myocardial substrates and found that myocardium obtained 70%, 15%, and 2% of carbon from free fatty acids, ketone bodies, and lactic acid, respectively. The basic metabolism involves many aspects such as tricarboxylic acid (TCA) cycle, oxidative phosphorylation, and high-energy phosphate metabolism. The heart can flexibly change metabolism according to the availability of substrates, with increased fatty acid levels decreasing glucose uptake, and vice versa [5]. Each metabolic substrate is not only a source of energy, but also an indispensable part of the metabolic pathway, which together play a role in the structural, functional, and metabolic restructuring of the heart.
Peroxisome proliferator activated receptors (PPARs) are fatty acid-activated transcription factors in the nuclear hormone receptor superfamily, which regulate energy metabolism and play an important regulatory role in glucose and lipid metabolism [6]. Currently, 3 PPAR subtypes have been identified: PPARα, PPARγ, and PPARβ/δ. PPAR is expressed in cardiovascular cells (endothelial cells, vascular smooth muscle cells, mononuclear/macrophages, etc.), and its ligands play an important role in various cardiovascular risk factors in clinical and preclinical studies, and PPAR is considered an important therapeutic target for atherosclerosis, inflammation, and hypertension [7]. More and more studies have found that myocardial metabolic abnormalities are closely related to the onset of numerous cardiovascular diseases. In 2004, Dutch scholar Bilsen [8] proposed the concept of cardiometabolic remodelling. Cardiomyocytes can be involved in the course of various cardiovascular diseases by affecting the original structure and systolic function of the heart through the imbalance of energy metabolism. Cardiometabolism is different from other organs in that it has multiple metabolic substances and can flexibly use various metabolic substrates to produce ATP, which reflects the characteristics of “omnivorous”. However, under the action of different pathological factors, cardiometabolism can develop into “biophagy”, which shows that cardiomyocytes will prefer a single metabolic substrate to obtain energy. Such a metabolic pattern will promote the disturbance of heart energy metabolism and insufficient energy supply, and eventually lead to the lack of ATP in cardiomyocytes, affecting the electrophysiological activities of the heart. More importantly, such a “bivoretic” metabolism in the long run will lead to excessive uptake of other metabolic substrates and deposit in cells to induce the structural restructuring of the heart. Thus, it mediates the occurrence of many cardiovascular diseases [6].
This study aims to explore the correlation between PPAR-α regulation of metabolic remodelling and myocardial fibrosis in rheumatic heart disease AF, including cardiac lipid metabolism, glucose metabolism, and mitochondrial functional metabolism indexes, and conduct verification analysis on myocardial fibroblasts. Finally, the correlation between PPAR-α regulation of metabolic remodelling and myocardial fibre in rheumatic heart disease AF was confirmed, which established the basis for further research on the mechanism of PPAR-α regulation of metabolic remodelling in AF myocardial fibrosis.
Material and methods
Left atrial appendage tissue and peripheral venous blood collection
In this study, patients with rheumatic heart disease complicated with valvular disease requiring thoracotomy were included as study objects (November 2021–November 2022). Patients with sinus heart disease complicated with valvular disease without AF required preventive resection of the left atrial ear during valve replacement; patients with AF included patients with rheumatic heart disease complicated with valvular disease and AF (Table I). During valve replacement, the left atrial appendage is removed to prevent thrombosis. Preoperative peripheral venous blood was collected from all the patients included in the clinical study, which was collected using a green head tube. After the collection, plasma was obtained by centrifugation at 3000 RPM and stored in a –80°C refrigerator in subpack for later ELISA index detection. Exclusion criteria were as follows: severe heart failure, hyperthyroidism, diabetes, and hyperlipidaemia patients. After obtaining the left atrial appendicular tissue, part of the tissue was fixed with 4% paraformaldehyde for later pathological section, and part of it was immediately stored in liquid nitrogen for protein extraction and WB detection. The PVDF membrane was incubated with primary antibodies specific for PPAR-α, GLUT4, CD36, PGC-1α, CPT-1b, PDK4 and SREBP-1 (1 : 1000), and GAPDH (1 : 5000). The quantitative analysis was achieved by detecting the grey value of protein bands. The volunteers signed the informed consent form, which met the ethical requirements.
Table I
Patient baseline data
Primary cultured cardiac fibroblasts and GW7647 intervention
Primary culture of SD rat myocardial fibroblasts was performed by the specific methods described above [9]. When the density of myocardial fibroblasts reached about 80%, the passage was carried out. They were divided into 3 groups: one group was a normal control group, one group was an ANG-II group (cell fibrosis model was established with a concentration of 10–5 µmol/l), and the other group was an ANG-II + GW7647 intervention group (The PPAR-α pathway cell agonist GW7647 and PBS solution were diluted into 1 ml agonist solution at the ratio of 1 : 100, and 30 µl agonist solution was added to 6-well plate). 24 h later, myocardial fibroblast immunofluorescence and myocardial fibroblast protein WB were detected.
Statistical processing
The data of each experimental group were described by means and standard deviation statistics, and the statistical software GraphPadPrism7.0 was used for analysis and processing. The statistical differences between the data of each experimental group were analysed by t-test and one-way variance (one-way ANOVA). When p < 0.05, the difference was considered statistically significant.
Results
Baseline data of included clinical study subjects
In this study, there were 30 patients in the AF group and the sinus group, and 7 patients in the sinus group did not meet the inclusion criteria. The baseline data of the 2 groups were basically consistent except for the factors caused by AF itself, such as left atrial diameter, left ventricular ejection fraction, and tricuspid valve regurgitation.
HE and Masson staining of left atrial appendage tissue sections
HE staining showed that, compared with the sinus group, the AF group had disordered myocardial tissue and abundant inflammatory cell infiltration. Masson staining showed that the myocardium in both the sinus group and the AF group had a certain degree of myocardial collagen infiltration, but the degree of myocardial fibrosis in the AF group was significantly higher than that in the sinus group (Figure 1).
Figure 1
Tissue section of the left atrial appendage stained by HE and Masson. A – HE and Masson staining of left auricle tissue sections showed that the left auricle of patients with AF was infiltrated with abundant inflammatory cells and that the cell arrangement was disordered. B – The proportion of collagen in the left auricular section between the 2 groups was compared (sinus group = 37.75±2.12%, AF group = 52.51 ±1.68%, p < 0.001). *vs. the control group, p < 0.05
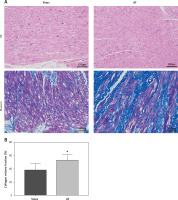
Plasma metabolism indexes were detected by peripheral venous ELISA
The collected peripheral venous blood of clinical patients was separated and the metabolic indexes in the plasma of each group were detected by ELISA kit. It was found that the levels of SOD, Malonyl COA, and ROS in the peripheral venous blood of patients in the AF group were significantly higher than those in the sinus group, with statistical differences (Figure 2).
Figure 2
Expression levels of plasma metabolic indices in the peripheral venous blood of clinical patients. A–C – Peripheral venous blood levels of SOD (sinus group = 3.861 ±0.5338 U/g, AF group = 5.383 ±0.4426 U/g, p = 0.0315), malonyl COA (sinus group = 0.3173 ±0.0368 ng/ml, malonyl COA), malonyl COA (sinus group = 0.3173 ±0.0368 ng/ml), malonyl COA (AF group = 3.861 ±0.5338 U/g, AF group = 5.383 ±0.4426 U/g, p =0.0315) AF group = 0.602 ±0.044 ng/ml, p = 0.003), and ROS (sinus group = 37.18 ±3.56 IU/ml, AF group = 56.7±4.121 IU/ml, p = 0.0012) were significantly higher than those in the sinus group. *vs. the control group, p < 0.05
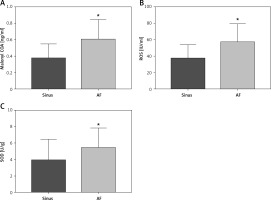
PPAR-α protein expression and metabolic index protein expression in left atrial appendage tissue
The left auricular tissue protein was extracted, and the expression of PPAR-α protein and metabolic indexes were detected by WB method. The indexes related to glucose metabolism were detected: GLUT4, PDK4; indexes of abnormal lipid metabolism CD36, SREBP-1; energy metabolism-related indexes: PGC-1α, CPT-1b, among which, the expression of PPAR-α, GLUT4, CD36, PGC-1α, and CPT-1b increased in the sinus group, and decreased in the AF group; and the expression of PDK4 and SREBP-1 decreased in the sinus group and increased in the AF group (Figure 3).
Figure 3
PPAR-α protein expression and metabolic index protein expression in left atrial appendage. A, B – Left atrial ear Western blotting strip and statistical results showed that GLUT4 expression levels were decreased in patients with AF (p < 0.05) and increased in patients with sinus (p < 0.05). PDK4 expression levels were decreased in patients with sinus (p < 0.05) and increased in patients with AF (p < 0.05). The expression levels of CD36 were decreased in the AF group (p < 0.05), and the expression levels of SREBP-1 were increased in the AF group (p < 0.05). For the energy metabolism-related indicators, PGC-1α expression was decreased in the AF group (p < 0.05), and CPT-1b expression was decreased (p < 0.05). *vs. the control group, p < 0.05. B – Left atrial ear Western blotting strip and statistical results showed that GLUT4 expression levels were decreased in patients with AF (p < 0.05) and increased in patients with sinus (p < 0.05). PDK4 expression levels were decreased in patients with sinus (p < 0.05) and increased in patients with AF (p < 0.05). The expression levels of CD36 were decreased in the AF group (p < 0.05), and the expression levels of SREBP-1 were increased in the AF group (p < 0.05). For the energy metabolism-related indicators, PGC-1α expression was decreased in the AF group (p < 0.05), and CPT-1b expression was decreased (p < 0.05). *vs. the control group, p < 0.05
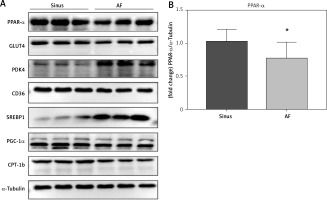
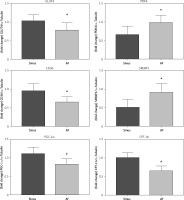
Correlation analysis between PPAR-α protein expression and fibrosis in left atrial appendage
Left auricular tissue protein was extracted from all clinical patients included in this time, and the expression of PPAR-α protein was detected by WB method. Correlation analysis was conducted between PPAR-α protein expression and the degree of fibrosis of corresponding specimens. Statistically, it was found that there was a negative correlation between PPAR-α protein expression and myocardial fibrosis (Figure 4).
Phenotypic identification of myocardial fibroblasts in primary culture SD rats
The cells selected in this study were myocardial fibroblasts of neonatal SD rats. Myocardial cells were isolated by differential adhesion method and stained by immunofluorescence. The myocardial fibroblasts could specifically express vimentin protein.
Phenotypic transformation of myocardial fibroblasts under the intervention of agonist GW7647
Myocardial fibroblasts undergo phenotypic transformation under the stimulation of various pathological factors and are transformed into myofibroblasts. α-SMA is a specific index to evaluate the phenotypic transformation of myocardial fibroblasts. After transformation, myofibroblasts increase their ability to synthesise and secrete collagen, and enhance their ability to promote myocardial fibrosis (Figure 5).
Figure 5
Phenotypic transformation of myocardial fibroblasts under intervention with the agonist GW7647. Myocardial fibroblasts underwent phenotypic transformation under the intervention of the PPAR-α agonist GW7647. Their ability to transform into myofibroblasts was significantly inhibited, and the expression of α-SMA was down-regulated (red fluorescence)
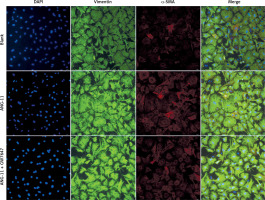
Collagen content of myocardial fibroblast supernatant
Collagen content in the cellular supernatant, including Collagen I and Collagen III, was detected by ELISA kit in cardiac fibroblasts under the intervention of PPAR-α agonists to evaluate the changes in collagen secretion level of cardiac fibroblasts under the intervention of PPAR-α agonists.
PPAR-α protein expression and metabolic index protein expression in myocardial fibroblasts
Collagen content in the cellular supernatant, including Collagen I and Collagen III, was detected by ELISA kit in cardiac fibroblasts under the intervention of PPAR-α agonists to evaluate the changes in collagen secretion level of cardiac fibroblasts under the intervention of PPAR-α agonists (Figures 6, 7).
Figure 6
Collagen content in the supernatant of cardiac fibroblasts. Collagen I (normal group = 0.754 ±0.064 μg/l, ANG-II = 1.322 ±0.05234 μg/l, p = 0.0024, Collagen I (normal group = 0.754 ±0.064 μg/l, Ang-II = 1.322 ±0.05234 μg/l, p = 0.0024), ANG-II + GW7647=0.930 ±0.117 μg/l, p =0.0378), Collagen III (normal group = 0.446 ±0.062 μg/l, ANG-II = 0.799 ±0.028 μg/l, p = 0.0069, Collagen III (normal group = 0.446 ±0.062 μg/l, Ang-II = 0.799 ±0.028 μg/l, p = 0.0069, ANG-II + GW7647 = 0.559 ±0.072 μg/l, p = 0.0373), *vs. between groups, n = 3, p < 0.05
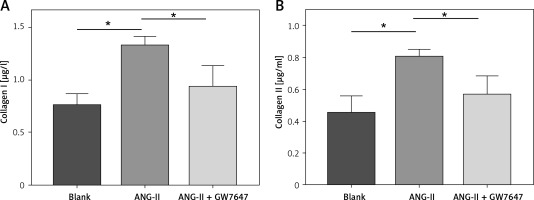
Figure 7
PPAR-α protein expression and metabolic index protein expression in myocardial fibroblasts. A, B – Cardiac fibroblast blot bands and statistical results of indicators related to glucose metabolism disorders. GLUT4 expression levels were decreased in the ANG-II group (p < 0.05) but increased in the GW7647 group (p < 0.05). PDK4 expression levels were decreased in the GW7647 group (p < 0.05) and increased in the ANG-II group (p < 0.05). The expression levels of CD36 were decreased in the ANG-II group (p < 0.05), and SREBP-1 expression levels were increased in the ANG-II group (p < 0.05). The expression levels of PGC-1α and CPT-1b were decreased in the ANG-II group (p < 0.05). *vs. the control group, n = 3, p < 0.05. B – Cardiac fibroblast blot bands and statistical results of indicators related to glucose metabolism disorders. GLUT4 expression levels were decreased in the ANG-II group (p < 0.05) but increased in the GW7647 group (p < 0.05). PDK4 expression levels were decreased in the GW7647 group (p < 0.05) and increased in the ANG-II group (p < 0.05). The expression levels of CD36 were decreased in the ANG-II group (p < 0.05), and SREBP-1 expression levels were increased in the ANG-II group (p < 0.05). The expression levels of PGC-1α and CPT-1b were decreased in the ANG-II group (p < 0.05). *vs. the control group, n = 3, p < 0.05
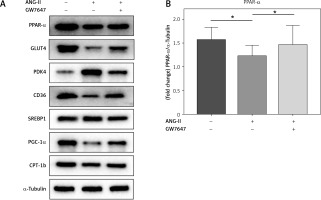
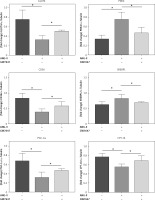
Discussion
In this study, we explored the correlation between PPAR-α regulation of metabolic remodelling and myocardial fibrosis in rheumatic heart disease AF. First, through the collection of clinical specimens, including left atrial ear tissue and peripheral venous blood, and pathological sections, we found that the myocardial inflammatory response of patients in the AF group was higher than that in the sinus group, and the myocardial inflammatory cell infiltration was abundant in the AF group. The myocardial fibre arrangement was disordered, and the degree of myocardial fibrosis in the AF group was significantly higher than that in the sinus group. Moreover, the degree of myocardial fibrosis in each AF patient was different. Is this different degree of myocardial fibrosis related to metabolism? We detected the expression of PPAR-α and the proteins related to the indexes of glucose metabolism, lipid metabolism, and energy metabolism by WB method. The results showed that the expression of PPAR-α in the left atrial ear tissue of patients with sinus disease was significantly up-regulated (p < 0.05), which was higher than that of patients with AF, indicating that PPAR-α negatively regulated myocardial fibrosis. Secondly, there were statistically significant differences in the expression of metabolism-related proteins (all p < 0.05), including GLUT4 and PDK4; the indexes of abnormal lipid metabolism were CD36 and SREBP-1; and the energy metabolism-related indicators were PGC-1α and CPT-1b. Among them, GLUT4, CD36, PGC-1α, and CPT-1b were decreased in patients with AF and increased in patients with sinus, playing a negative regulatory role; PDK4, SREBP-1 were increased in patients with AF and decreased in patients with sinus, playing a positive regulatory role. In addition, in peripheral venous blood, ROS, SOD, and malonyl-COA, which are related to mitochondrial energy metabolism and oxidative stress, were significantly increased in peripheral blood of patients with AF (all p < 0.05), indicating that mitochondrial energy metabolism and oxidative stress response in myocardial tissue were enhanced during AF. We performed a correlation analysis between PPAR-α protein expression and myocardial fibrosis and found that there was a statistically negative correlation between PPAR-α protein expression and the degree of myocardial fibrosis (r = –0.5322, p < 0.0001). To further confirm the correlation between PPAR-α regulation of metabolic remodelling and myocardial fibrosis, we cultured cultured neonatal SD rat myocardial fibroblasts and stimulated them with PPAR-α agonist GW7647 to detect the effect of GW7647 on the phenotypic transformation of myocardial fibroblasts. The results showed that, after GW7647 stimulation, the ability of myocardial fibroblasts to transform into myofibroblasts was significantly inhibited, and collagen secretion levels (Collagen I and Collagen III) in the supernatant of fibroblasts were significantly decreased after GW7647 stimulation (all p < 0.05). These results indicated that the activation of PPAR-α by GW7647 could inhibit the ability of myocardial fibroblasts to secrete collagen and reduce myocardial fibrosis.
The heart uses different carbon substrates to produce energy, and although the adult heart primarily consumes fatty acids, it shows flexibility in the use of energy types, being able to metabolise glucose, lactic acid, fatty acids, and ketone bodies, the heart uses nutrients to maintain muscle activity and anabolic responses. For example, physiological heart growth (cardiomyocyte proliferation) and pathological heart remodelling (cardiomyocyte hypertrophy) in the foetus [6]. In cardiac energy metabolism, PPARs, a fatty acid-activated transcription factor in the nuclear hormone receptor superfamily, regulates energy metabolism, plays an important regulatory role in glucose and lipid metabolism, and is the master switch regulating the enzymatic mechanism of cardiomyocytes, in which the activation of PPAR-α (e.g. by betate drugs) normalises atherosclerotic dyslipidaemia, thereby reducing the risk of cardiovascular disease [10, 11]. Activation of PPAR-α and PPAR-δ signalling, especially in the early stages of cardiac remodelling, may be a promising treatment strategy for heart failure [12, 13] because the preferred energy substrate used by the heart changes from fatty acids to glucose and the expression of PPAR-α and PPAR-δ pathways is inhibited during heart failure or stress. In this study, we explored the correlation between PPAR-α regulation of metabolic remodelling and myocardial fibrosis in rheumatic heart disease AF. Previous studies on PPAR-α and myocardial fibrosis were few. In a rat hypertensive model induced by deoxycorticosterone acetate, PPAR-α activation can reduce myocardial fibrosis and prevent the development of diastolic dysfunction. The possible mechanism of action is mediated by prevention of inflammatory mediators through NF-κB pathway [13]. In a rat model of hypertensive myocardial fibrosis induced by angiotensin II, the PPAR-α activator fenofibrate prevents the progression of hypertension and improves myocardial inflammation and collagen deposition in rats [14]. In this study, we found that there was a correlation between PPAR-α and myocardial fibrosis in AF, and there were statistical differences in the protein expressions of glucose metabolism, lipid metabolism, and energy metabolism indexes regulated by PPAR-α (all p < 0.05). These results indicate that glucose metabolism, lipid metabolism and energy metabolism are involved in the mechanism of PPAR-α regulation of myocardial fibrosis.
GLUT4, a glucose transporter, is mainly expressed in myocardial tissue and skeletal muscle tissue, and it is the main protein that determines glucose uptake in myocardial tissue. We found that GLUT4 was down-regulated in patients with AF, indicating that myocardial glucose uptake decreased and the proportion of myocardial metabolic substrate changed during AF. PDK4 is pyruvate dehydrogenase kinase 4, which can promote the phosphorylation of pyruvate dehydrogenase, thereby inhibiting glucose metabolism [15]. The expression of PDK4 was elevated in the AF group, which also indicates that glucose metabolism is inhibited during AF. CD36 is a leukocyte differentiation antigen 36; its main function is to clear low-density lipoprotein, and its main function in myocardium is the intake of free fatty acids. The expression of CD36 decreased in AF, indicating that the metabolism of fatty acids in myocardial tissue decreased in AF. SREBP-1 is a cholesterol-regulating element binding protein 1, which promotes the synthesis of triglycerides and other ester compounds, and it promotes the aggregation of lipids in cells, resulting in cytotoxicity. The expression of SREBP-1 was increased in the AF group, and combined with the expression of CD36, it indicates that the level of myocardial lipid metabolism is decreased during AF. Previous studies have confirmed that the decrease of lipid metabolism in myocardial tissue leads to lipid accumulation, which leads to myocardial fibrosis and promotes myocardial remodelling [16]. PGC-1α is a peroxisome proliferator activating receptor γ-coactivator 1α, mainly distributed in myocardial tissue, skeletal muscle, liver, and other tissues, mainly involved in mitochondrial metabolic pathways, and is closely related to cardiovascular diseases [17], which has been confirmed by studies. Fenofibrate inhibits myocardial metabolic remodelling by regulating the PPAR-α/sirtuin 1/PGC-1α pathway [18]. CPT-1b, an isomer of carnitine palmitoyl transferase 1b, is mainly responsible for promoting the transfer of long-chain ester acyl-coenzyme A into mitochondria for oxidative stress reaction, which affects the rate of lipid metabolism in myocardial tissue. CPT-1b expression is down-regulated in the AF group [19], indicating that AF not only affects energy metabolism in myocardial tissue, but also lipid metabolism in myocardial tissue.
In conclusion, we came to the following conclusions: (1) PPAR-α expression is negatively regulated in the left atrial appendage of patients with rheumatic heart disease and plays a protective role. (2) PPAR-α expression was negatively correlated with the degree of left atrial fibrosis in patients with rheumatic heart disease. (3) PPAR-α activation can inhibit the phenotypic transformation of myocardial fibroblasts into myofibroblasts, reduce collagen secretion, and improve myocardial fibrosis. Regulating the expression of PPAR-α is expected to improve myocardial fibrosis and provide a new strategy for the treatment of AF. Of course, this study also has certain limitations, First, the number of clinical samples included in this study is small, so the conclusion has certain limitations; second, the specific mechanism of action of PPAR-α in regulating metabolic remodelling and participating in myocardial fibrosis of AF needs further research.