Introduction
Osteoporosis is a common bone disorder affecting over 2 billion people throughout the world [1]. It is characterised by decreased bone density, increased bone fragility, and changes in bone structure [2]. Post-menopausal osteoporosis also results in an increased incidence of bone fractures [3]. In China, 40.1% of women over the age of 50 years suffer from osteoporosis [4]. Osteoporosis alters bone remodelling by decreasing osteoblast formation and increasing osteoclastic bone reabsorption [5]. In addition, multinucleated osteoclasts form due to the binding of haematopoietic monocytes to osteoclast precursors, activating the receptor activator of nuclear factor-κB ligand (RANKL) signalling pathway [6]. This enhances the process of osteoclastogenesis [7]. Due to an interaction between RANKL and its receptor RANK, TNF receptor-associated factor 6 (TRAF6) is recruited and upregulates several osteoclast genes, such as DPD, cathepsin, and TRAP [8]. In addition, nuclear factor of activated T cell c1 (NFATc1) is induced by NF-κB and is reported to regulate the activation, fusion, and differentiation of RANKL-induced osteoclasts [9].
Traditional drugs used for the management of postmenopausal osteoporosis have several undesirable side effects including osteonecrosis [10]. During the last few decades, molecules from natural sources have shown beneficial results in the management of chronic disorders. Pristimerin is a triterpenoid isolated from plants in the family Celastraceae [11]. These plants are used in traditional Chinese medicine. Pristimerin has been used for the treatment of inflammatory and autoimmune disorders as well as tumours [12]. It also has the ability to inhibit inducible nitric oxide synthase (iNOS) and reduce NOx [13], which results in anti-inflammatory activity and a hepatoprotective effect [14]. In addition, it inhibits NF-κB and its resulting inflammatory cascade [15], and has a protective effect against arthritis by modulating the RANKL/OPG pathway and reducing inflammatory mediators [12]. We evaluated its protective effects against osteoporosis in ovariectomised rats.
Material and methods
Animals
Female Wistar rats were procured from Shanghai Medical College, Shanghai, China. All animals were stored under standard conditions with a 7-day acclimatisation period and free access to a standard chow diet and tap water. All protocols in this study were approved by the Institutional Animal Ethical Committee of Shanghai JiaoTong University, Shanghai, China (SJTU/IAEC/2017/07).
Chemicals
Pristimerin was provided by Sigma Aldrich Ltd., USA, and alendronate was provided by Hangzhou Moshadong Pharmaceutical Co. Ltd., China. ELISA kits with collagen type I fragments, osteocalcin, cathepsin K, BGP, TNF-α, and IL-1β were purchased from Thermo Fisher Scientific Ltd., USA. Antibodies were procured from Bio-Techne China Ltd., China.
Procedure
All animals were randomly separated into two different groups. Ovaries were removed from the ovariectomy group after anesthetisation with pentobarbital (40 mg/kg, i.p.). The sham group (n = 10) underwent surgery without removing the ovaries. After 3 days of recovery, the ovariectomy group was further separated into five groups (each group n = 10). Negative controls received saline solution, the standard treatment group received alendronate (2.5 mg/kg), and the three pristimerin groups received 50, 100, and 200 mg/kg over a 4-week period.
Measurement of biochemical parameters
The plasma concentration of alkaline phosphatase (ALP) was estimated using a colorimetric assay kit. An ELISA kit was used to determine the serum concentration of collagen type I fragments (CTX) and osteocalcin (OC) protein. The levels of cathepsin K, BGP, TNF-α, and IL-1β in serum were estimated using their respective kits. The activity of tartrate-resistant acid phosphatase (TRAP) was estimated using a p-nitrophenyl sodium phosphate assay.
Measurement of bone microarchitecture
Micro computed tomography (CT) analyses were performed as previously reported [16]. Scans were used to determine the microarchitecture of trabecular bone tissue from the distal right femur. ABA bone analysis software was used to estimate bone morphometric parameters at the growth plate such as trabecular thickness, number of trabeculae, trabecular separation, structure model index, ratio of trabeculae to total bone volume, and bone mineral density.
Western blotting assay
Protein was extracted from the right tibia and the lysate was centrifuged at 12,000 RPM for 10 min to obtain a supernatant. A bicinchoninic acid quantification assay was used to estimate protein concentration. Proteins were separated via 10% SDS-PAGE and then transferred to a polyvinylidene difluoride membrane. The membrane was further incubated with primary antibodies including Akt, PI3K, RANKL, NF-κB, p65, TRAF-6, and β-actin. Later, the membrane was washed and the chemiluminescent signals of the blots were imaged.
Cell culture
RAW 264.7 cells were procured from the Cancer Research Center, Shanghai, China. Dulbecco’s modified Eagle medium (DMEM) supplemented with streptomycin (100 μg/ml), penicillin (100 U/ml), and FBS (10%) was used to culture the cell line in 5% CO2 at 37°C.
MTT assay
The viability of RAW264.7 cells was estimated using a 3-(4,5-dimethylthiazol-2-yl)-2,5-diphenyl tetrazolium bromide (MTT) colorimetric assay. Cells (3 × 104 cells/well) were seeded in a 24-well plate and incubated with pristimerin (5, 10, 25, and 50 μM) overnight. The following day, the cells were incubated with MTT for 4 h. A FlexStation 3 Multi-Mode Microplate Reader was used to detect absorbance at a wavelength of 492 nm.
Osteoclastogenesis assay
RAW 264.7 cells were seeded into a 24-well plate (1 × 106 cells/well) and cultured with RANKL (50 ng/ml) and pristimerin (5, 10, 25 and 50 μM). A TRAP staining kit was used to measure the formation of osteoclasts. TRAP-positive, mature osteoclasts were counted using a light microscope.
Immunofluorescent staining
RAW 264.7 cells were seeded in a 24-well plate (1 × 106 cells/well) and incubated overnight. They were later treated with pristimerin (5, 10, 25, and 50 μM) for 2 h and further stimulated with RANKL (50 ng/ml) for 1 h. Thereafter, the cells were washed with PBS twice and treated with paraformaldehyde (4%) at room temperature for 15 min to fix the cells. Anti-p65 antibody (1 : 200) was incubated with the cells overnight at 4°C and then Alexa Fluor 488 phallotoxin (1 : 500) was used at room temperature for 1 h to label the cells. Cells were washed with PBS and further stained with 4′-6-diamidino-2-phenylindole (DAPI) at room temperature for 3 min. Confocal microscopy was used to visualise the immunohistochemical changes.
Reabsorption pit assay
RAW 264.7 cells were seeded in a 24-well plate (2000 cells/well) and incubated for 7 days with pristimerin (5, 10, 25, and 50 μM) and RANKL (50 ng/ml). The medium was changed every 3 days. The cells were treated overnight with ammonium hydroxide (1 M) to remove the attached cells, which then were placed on bone slices. The number of pits that form indicate the level bone reabsorption, which is related to the activity of osteoclasts. Image Pro-Plus software (v. 4.0) was used to count the reabsorption pits.
Results
Effect of pristimerin on bone formation
The effects of pristimerin on markers of bone formation such as CK, ALP, TRAP, CTX, BGP, and OC in the serum of ovariectomised rats are shown in Figure 1. There was a significant increase in the levels of CK, ALP, TRAP, CTX, and BGP and a significant decrease in the level of OC in the serum of negative controls compared to controls. Treatment with pristimerin significantly attenuated the altered level of these biochemical markers.
Effect of pristimerin on cytokine production
The effects pristimerin on the level of inflammatory cytokines such as IL-1β and TNF-α are shown in Figure 2. There was a significant decrease in the level of inflammatory cytokines in the pristimerin and standard treatment group compared to negative controls.
Effect of pristimerin on bone microarchitecture
Figure 3 shows the effects of pristimerin on bone microarchitecture. There was a significant (p < 0.01) decrease in BMD tubular number, tubular thickness, and BV/TV in the bone tissues of negative controls compared to controls. However, SIM and tubular separation in the bone tissue increased in negative controls compared to controls. Treatment with alendronate and pristimerin attenuated the changes in markers of bone microarchitecture such as BMD, Tb.N. Tb.Th, Tb.Sp, SIM, and BV/TV.
Effect of pristimerin on the expressions of Akt, PI3K, RANKL, NF-κB p65, and TRAF-6
The effects of pristimerin on the expression levels of Akt, PI3K, RANKL, NF-κB p65, and TRAF-6 in bone tissue are shown in Figure 4. The expression levels of TRAF-6, NF-κB p65, and RANKL were significantly increased (p < 0.01), and the expression levels of Akt and PI3K were significantly reduced, in negative controls compared to the sham group. Treatment with pristimerin significantly reversed both trends.
Effect of pristimerin on viability of cells in vitro
Figure 5 shows the results of an MTT assay to assess the viability of RAW 264.7 in the presence of pristimerin. RAW 264.7 cells treated with pristimerin at 5, 10, 25, and 50 μM exhibited 100.1%, 99.4%, 98.39% and 99.0%, viability, respectively. Thus the pristimerin groups show no increase in toxicity compared to the other groups.
Effect of pristimerin on differentiation of osteoclasts
Figure 6 shows the effects of pristimerin on osteoclast differentiation. RANKL increased the formation of osteoclasts in RAW264.7 cells and treatment with pristimerin attenuated this effect.
Figure 6
Effect of pristimerin on differentiation of osteoclasts. A – TRAP-positive multinucleated mature osteoclast cells under a light microscope. B – Number of TRAP-positive osteoclast cells
Mean ± SEM (n = 10), ##p < 0.01 compared to sham group; *p < 0.05, **p < 0.01 compared to negative controls.
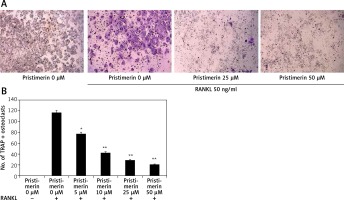
Effect of pristimerin on localisation of p65
Stimulation with RANKL localised p65 to the nucleus and pristimerin blocked this effect (Figure 7).
Effect of pristimerin on bone reabsorption
Treatment with pristimerin significantly (p < 0.01) reduced the amount of bone reabsorbed by RANKL-stimulated RAW264.7 cells compared to the RANKL-only group (Figure 8).
Discussion
Osteoporosis is the most common bone disorder affecting post-menopausal women. Current treatment options for osteoporosis have several limitations [2]. There are several markers of bone formation and reabsorption such as ALP, CTX, OC, BGP, and TRAP [17]. Alendronate is the firstline drug for treating osteoporosis and has been shown to restore normal levels of these markers [18]. Our results indicate that treatment with pristimerin has a similar outcome as treatment with alendronate. Menopause causes a deficiency in oestrogen, which is associated with an increase in the level of inflammatory cytokines [19–21]. Treatment with pristimerin significantly (p < 0.01) reduced the level of inflammatory cytokines in oestrogen-deficient mice.
The integrity of the skeletal system is maintained by coordination of the activity between osteoclasts and osteoblasts [22]. RANKL stimulates osteoclastogenesis, increasing bone reabsorption [23]; membrane-bound protein p65, resulting in translocation to the nucleus [24]; and the PI3K and NF-κB pathway, enhancing osteoclastogenic activity [25]. Our data reveal that treatment with pristimerin restores the normal activity of PI3K, NF-κB, and p65 in RANKL-stimulated bone tissue of ovariectomised rats.
TRAF6 is a novel target for the management of osteoporosis [26]. Research has shown that TRAF-6-deficient transgenic mice suffer from osteoporosis and exhibit increased osteoclastogenesis [27]. RANKL is thought to downregulate TRAF-6. Our results suggest that pristimerin treatment restores the normal expression of TRAF-6 in ovariectomised rats.
To further confirm the effects of pristimerin on the RANKL signalling cascade, we performed in vitro analyses using RAW 264.7 cells. First, we evaluated the cytotoxic effects of pristimerin on RAW 264.7 cells and found that there were no negative effects on cell viability. Next, we examined TRAP, which is an enzyme released in bone tissue by osteoclastic activity. RANKL increased the number of TRAP-positive cells and treatment with pristimerin significantly reduced their number. In addition, treatment with pristimerin blocked RANKL-stimulated translocation of p65 to the nucleus and reduced the number of pits in RAW 264.7 cells. This indicates that pristimerin affects the RANKL signalling cascade and bone reabsorption caused by the translocation of p65 in our in vitro model.
In conclusion, pristimerin effectively treats osteoporosis by reducing stimulation of the RANKL signalling pathway and restoring normal levels of TRAF-6 and NF-κB, thereby reducing osteoclastic activity in bone tissue.