Introduction
Thyroid cancer is typically diagnosed with 5–10% of the whole thyroid nodule and is classified according to the cell origin and development stage. Papillary thyroid cancer (PTC), follicular thyroid cancer (FTC), poorly differentiated thyroid cancer and undifferentiated thyroid cancer (anaplastic thyroid cancer) derive from follicular cells, while medullary thyroid cancer, lymphadenoid goitre, and metastatic cancer of the thyroid gland originate from non-follicular cells [1–3]. PTC is the most common cancer in middle-aged women and composes over 60% of all thyroid cancers [4–7]. PTC is known to be involved in the activation of the tyrosine kinase pathway, which is one of the typical cell signalling pathways [8]. A previous study reported that several genetic factors, such as rearrangement during transfection (RET)/PTC rearrangement [9–11] and mutation of RAS [12, 13] and BRAF [9, 14–17], cause PTC to occur. These genes are exclusively related to each other. However, the next factor and exact molecular mechanism underlying the progression of thyroid cancer remain unclear. FTC frequently occurs when there is a deficiency of iodine and the RAS mutant and rearrangement of the PAX8-PPARy gene are found [18]. The occurrence and progression of thyroid cancer involve a number of factors, especially thyroid-stimulating hormone (TSH), which is known to play an important role. TSH not only drives the growth of thyroid cells but also stimulates the growth of differentiated thyroid cancer [19–21]. In addition, TSH plays an important role in supplying oxygen and nutrients through peripheral blood vessels in cancer cells and is involved in a number of growth factors, such as fibroblast growth factor (FGF) [22–26], insulin-like growth factor-1 (IGF-1) [22, 26, 27], transforming growth factor-α (TGF-α) [22, 26], angiogenesis factor, and vascular endothelial growth factor (VEGF) [22, 26, 28–30].
MicroRNAs (miRNAs) are small non-coding RNAs of 19–24 nucleotides that control the translation and gene expression of target mRNA by binding to regulatory sites [31]. There is much evidence that miRNAs play important roles in various biological processes, including cell proliferation, metabolism, differentiation, development and apoptosis [32, 33]. Many studies have already examined the association between miRNA expression and various types of cancer. Aberrant expression of miRNA is known to be correlated with important processes during carcinogenesis [33, 34]. Chen et al. demonstrated that miR-524-5p was associated with the overall survival rate and pathological grade of glioma patients [35], and Liu et al. reported that miR-524-5p could inhibit migration and proliferation through the MAPK signalling pathway in melanoma cells [36]. However, the role of miR-524-5p in thyroid cancer-related mechanisms is not clear.
Ginsenoside Rh2 is known as a saponin of ginseng and one of the ginsenoside families, which include Rb1, Rd, Rg1-3, Rh1, Rh2, and compound K (CK). Rh1, Rh2 and CK have high absorption rates in the body. Rh2 has a low molecular weight and low toxicity and many merits for health, including antioxidant, anti-inflammatory and anticancer effects [37, 38]. Numerous studies have reported that Rh2 induces apoptosis and regulates immune function in human cancer cells by blocking cell proliferation as well as inhibiting metastasis and angiogenesis [39]. Previous studies have shown that Rh2 can induce apoptosis by activating the death receptor TRAIL-R1 (DR4) signalling pathway [40]. However, it is still unclear whether Rh2 affects thyroid cancer.
In this investigation, we evaluated the considerable change in the expression level of miR-524-5p in the thyroid cancer cell line K1 as well as the effect of treatment with Rh2. Moreover, we demonstrated the relationship between Rh2 and miR-524-5p in thyroid cancer cells. Rh2 and the miR-524-5p mimic, or inhibitor, were introduced to thyroid cancer cells to evaluate their roles in cell migration and proliferation. Our data will be useful for understanding the function of miR-524-5p and mechanism of Rh2 in thyroid cancer cell development.
Material and methods
Cell culture and treatment of Rh2
TPC-1, K1, and NPA human thyroid cancer cell lines as well as the normal thyroid cell line Nthyri3-1 were purchased from the American Type Culture Collection (Manassas, VA, USA). All cells were cultured in PRMI1640 (Gibco, USA) containing 10% heat-inactivated FBS (Thermo Fisher), 1% streptomycin, and 1% penicillin (Sigma-Aldrich) in an incubator supplying 5% CO2 at 37°C. Cultured K1 cells were treated with 50 μM Rh2.
RNA extraction and RT-qPCR analysis
Total RNA was extracted from the cell lines using Invitrogen TRIzol reagent (Thermo Fisher Scientific, Inc.). cDNA was synthesized using the PrimeScript RT reagent Kit (Takara Biotechnology Co., Ltd., Dalian, China) using RNA as a template according to the manufacturer’s instructions. gDNA contamination was erased by RNase-free DNase (Promega, MA, USA). The expression of miR-524-5p was detected with specific primers, and GAPDH was used as an internal control. Relative expression levels were calculated using the ΔΔ Ct method.
Western blot analysis
After incubation of the cell lines, the whole cells were washed twice with 1X PBS and harvested. Then, 200 μl of RIPA buffer (Sigma, USA) containing protease inhibitor cocktail was added to the cells. After lysis and centrifugation, the protein concentration was measured. Twenty micrograms of protein from each sample was separated on 10% SDS-PAGE gels and further transferred onto PVDF membranes. The membranes were incubated with primary antibodies and further treated with the corresponding secondary antibody. The signal was visualized with an enzyme-linked chemiluminescence kit (Beyotime Institute of Biotechnology, Shanghai, China). The band density was quantified by ImageJ software (NIH) and normalized to that of GAPDH.
Cell proliferation assay
Cultured cells were treated with 50 μM Rh2, transfected with 10 nM miRNA or treated with miR-524-5p inhibitor and seeded in 96-well plates. After 24 h of incubation, cell density was examined with an MTT assay kit (Invitrogen, USA) according to the manufacturer’s instructions. The cell density was determined by measuring the absorbance at 490 nm. The experiments were repeated at least three times.
Results
Expression level of miR-524-5p in human thyroid cancer cell lines
miR-524-5p was reported to play an important role in the proliferation and migration of different types of cancer cells; however, its role in thyroid cancer has not been reported. To analyse miR-524-5p function, its expression level was analysed in the normal thyroid cell line Nthyri3-1 and the thyroid cancer cell lines TPC-1, K1, and NPA. Interestingly, the expression levels of miR-524-5p were significantly decreased in TPC-1, K1, and NPA compared to Nthyri3-1. The miR-5245p level was almost 4-fold lower in thyroid cancer cell lines compared to normal thyroid cell lines, while the level was similar between the three thyroid cancer cell lines TPC-1, K1, and NPA (Figure 1).
Figure 1
miR-524-5p expression levels in human thyroid cancer cell lines. miR-524-5p expression levels in human thyroid cancer cell lines TPC-1, K1, and NPA, and normal thyroid cell line Nthyri3-1 were examined by qRT-PCR. Significant differences between thyroid cancer cells and Nthyri3-1 cells are shown (**p < 0.01). The error bars represent the mean ± SE (n = 3)
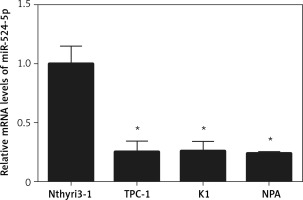
Ginsenoside Rh2 treatment inhibits K1 thyroid cell migration and proliferation
Ginsenoside Rh2 is known as an anticancer molecule used for cancer therapy. To analyse the effect of Rh2 on thyroid cancer cells, the K1 cell line was treated with 50 μM Rh2, and the migration and proliferation of thyroid cells were examined. After 24 and 48 h of Rh2 treatment, cell migration was monitored. Compared to the untreated control, Rh2 application significantly inhibited cell migration speed of K1 cells at the 24- and 48-hour time points (Figure 2 A). In addition, K1 cell proliferation was monitored via the MTT assay. Similar to the migration rate, 50 μM Rh2 treatment significantly inhibited cell proliferation, as determined by analysing the OD value at 490 nm (Figure 2 B).
Figure 2
Rh2 treatment inhibits K1 thyroid cancer cell migration and proliferation. A – Cell scratch assay was performed to test effects of Rh2 on K1 thyroid cancer migration. The cell migration distance at 24 and 48 h after 50 μM Rh2 treatment was measured. B – Cell proliferation was measured via MTT assay
Data represent mean values ± SE of 20 replicates, as compared to the control group (*p < 0.05).
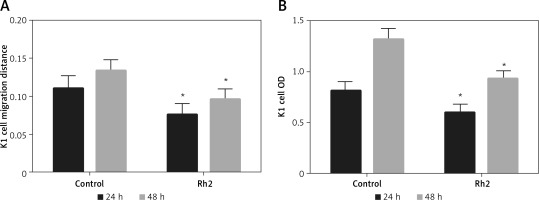
Ginsenoside Rh2 treatment induces miR-524-5p expression
Ginsenoside Rh2 treatment inhibited K1 thyroid cancer cell migration and proliferation, and miR-524-5p was reported to positively regulate other cancer cell migration and proliferation. However, miR-524-5p expression levels were lower in thyroid cancer cell lines than in normal thyroid cells. To examine whether Rh2 can regulate miR-524-5p, K1 cells were treated with 50 μM Rh2, and the expression levels of miR-524-5p were monitored by qRT-PCR after 24 h of Rh2 treatment. The results indicated that Rh2 treatment induced miR-524-5p expression approximately 3.5-fold compared to untreated control cells (Figure 3 A).
Figure 3
Effects of Rh2 on miR-524-5p level and overexpression of miR-524-5p on thyroid cancer cell regulating protein levels. A – miR-524-5p level was examined after 48 h of Rh2 treatment. B – miR-524-5p levels in miR-control and miR-524-5p overexpressed cells. C – Western blot analysis was performed to analyze E-cadherin and vimentin levels after Rh2 treatment or miR-524-5p overexpression. GAPDH was used as an internal control. Control: without Rh2 treatment. D – The relative band densities shown in (C) were measured using ImageJ software
Data represent mean values ± SE (n = 3). Significant differences between control and Rh2 treated groups or miR-control and miR-524-5p overexpressed groups are indicated (*p < 0.05; **p < 0.01).
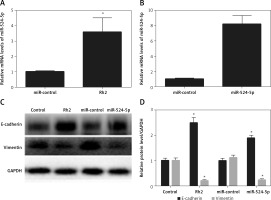
The miR-524-5p mimic exhibited similar effects to those of Rh2 treatment Since miR-524-5p levels were lower in thyroid cancer cells, miR-524-5p was overexpressed to analyse its function. The miR-524-5p mimic was expressed in K1 thyroid cancer cells, and qRT-PCR results showed that miR-524-5p expression levels were approximately 8-fold higher than those in non-transformed cells (Figure 3 B). E-cadherin and vimentin are marker proteins used to examine cancer cell status. To understand the effect of Rh2 and the miR-524-5p mimic on marker protein levels, western blot analysis was performed. Rh2-treated cells and miR-524-5p mimic-expressing cells showed similar marker protein levels. The E-cadherin level was higher in both Rh2-treated and miR-524-5p mimic-expressing cells, while the vimentin level was lower in Rh2-treated and miR-524-5p mimic-expressing cells than in the control cells (Figures 3 C, D).
The miR-524-5p mimic inhibited K1 thyroid cancer cell migration and proliferation
Since E-cadherin and vimentin levels were similar in Rh2-treated and miR-524-5p mimic-expressing cells, cell migration and proliferation were analysed in miR-524-5p mimic-expressing K1 thyroid cancer cells. miR-524-5p mimic expression delayed cell migration speed after 24 and 48 h of scratch generation (Figure 4 A). Additionally, the cell proliferation rate was also reduced in miR-524-5p mimic-expressing cells after 24 and 48 h of transformation (Figure 4 B).
Figure 4
Effect of overexpression of miR-524-5p on migration and proliferation of K1 thyroid cancer. A – Cell scratch assay was performed to test effects of miR-524-5p on K1 thyroid cancer migration. Cell migration distance at 24 and 48 h. B – Cell proliferation was measured before via MTT assay
Data represent mean values ± SE of 20 replicates, as compared to the control group (*p < 0.05).
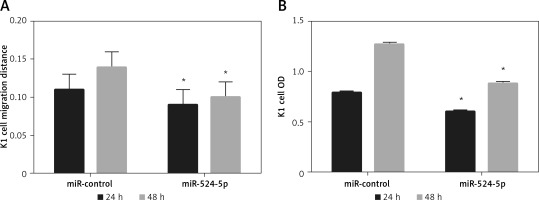
miR-524-5p inhibition alleviated Rh2 action on K1 thyroid cell migration and proliferation
Ginsenoside Rh2 inhibited thyroid cell migration and proliferation and induced the expression of miR-524-5p. In addition, miR-524-5p mimic expression inhibited cell migration and proliferation; therefore, we further examined miR-524-5p function during Rh2-mediated inhibition of K1 thyroid cancer cell migration. Rh2 treatment inhibited cell migration, but inhibition of miR-524-5p in Rh2-treated cells reduced the cell migration speed. The migration rate was similar in control cells after 24 and 48 h of scratch generation (Figure 5 A). Additionally, cell proliferation was inhibited by Rh2 treatment, and miR-524-5p inhibition eliminated Rh2 action (Figure 5 B).
Figure 5
Action of miR-524-5p inhibitor on migration and proliferation of K1 thyroid cancer cells under Rh2 treatment condition. A – Cell scratch assay was performed to test effects of miR-524-5p on K1 thyroid cancer migration with Rh2 stimuli. Cell migration distance at 24 and 48 h. B – Cell proliferation was measured before via MTT assay
Data represent mean values ± SE of 20 replicates, as compared to the control group (*p < 0.05).
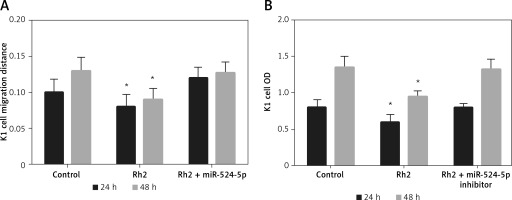
Discussion
Panax ginseng, which is the most common species of ginseng, is widely used in Asian countries and has many benefits for human health, as it exhibits anti-inflammatory, antioxidant and anticancer effects [41]. Ginseng saponins, usually called ginsenosides, are emphasized in cancer chemoprevention and therapeutics [37]. Numerous studies have investigated whether ginseng induces apoptosis in mammalian tumour cells by blocking cell proliferation [38]. Ginsenoside Rh2 has the characteristics of low toxicity, a low molecular weight and good fat solubility. It has been proven that it can inhibit proliferation, the transfer of tumour cells, and angiogenesis. However, Rh2 function in thyroid cancer therapy has not been reported. Extensive studies revealed that miRNAs are involved in different types of human cancer development, and normally, the expression levels of miRNAs are different from adjacent cells in cancer tissues; therefore, many researchers think that miRNAs might act as oncogenes or suppressors [42, 43].
In this study, we examined miR-524-5p and ginsenoside Rh2 function in thyroid cancer cells. Ginsenoside Rh2 has been known to be an anticancer molecule, and in our analyses, Rh2 treatment of the thyroid cancer cell line K1 inhibited the proliferation and migration of K1 cells. E-cadherin and vimentin are marker proteins of cancer cells, and E-cadherin levels are negatively associated with cancer cell migration and proliferation, while vimentin levels are positively associated with cancer cell migration and proliferation. The western blot analysis data showed that Rh2 treatment evidently induced E-cadherin while suppressing vimentin levels, suggesting that the phenomena of K1 cell migration and proliferation are associated with marker protein levels. These data also indicate that ginsenoside Rh2 may inhibit cancer. Interestingly, Rh2 treatment induced miR-524-5P. miR-524-5p is a type of miRNA, and its function was analysed in other cell types, e.g., gastric cancer, indicating that miR-524-5p negatively regulates cancer cell migration, proliferation and apoptosis. To analyse miR-524-5p function in thyroid cancer cells, the miR-524-5p expression levels were analysed in normal thyroid cell and cancer cell lines. The data showed that miR-524-5p expression levels were obviously lower in the three thyroid cancer cell lines than in the normal thyroid cancer cells. Further examination using overexpression (mimic) of miR-524-5p demonstrated that miR-524-5p negatively regulates K1 thyroid cancer cell migration and proliferation. In other words, overexpression of miR-524-5p inhibited K1 cell migration and proliferation. Additionally, the cancer cell marker protein E-cadherin and vimentin levels were higher and lower, respectively, in miR-524-5p-overexpressing K1 cells than in control cells, which further confirmed cancer cell status at the molecular level, suggesting that the delays of cell migration and proliferation were matched with E-cadherin and vimentin levels.
The effects of Rh2 and miR-524-5p overexpression on thyroid cancer cell migration and proliferation were similar. Furthermore, Rh2 and miR-524-5p mimic induced E-cadherin while suppressing vimentin levels in the thyroid cancer cell line K1, and Rh2 treatment induced miR-524-5p expression. Therefore, the question that arises is whether Rh2 inhibits thyroid cancer cell migration and proliferation via activation of miR-524-5p. To address this question, the thyroid cancer cell line K1 was cotreated with Rh2 and miR-524-5p inhibitor. The data showed that Rh2-mediated inhibition of K1 cell migration and proliferation was restored by miR-524-5p inhibitor, suggesting that Rh2 action requires miR-524-5p induction in thyroid cancer cells.
In conclusion, our analyses identified that ginsenoside Rh2 inhibits thyroid cancer cell migration and proliferation possibly via regulation of miR-524-5p. The results provided here might be useful for further understanding of ginsenoside Rh2 anticancer mechanisms and miRNA functions in thyroid cancer development.