Introduction
Autologous saphenous vein and internal mammary artery grafting have been broadly used as bypass conduits during coronary artery bypass graft (CABG) surgery. The success of surgery depends on the long-term patency of the conduits. Vasospasm of the arterial and venous grafts is a significant clinical problem, it can contribute to the development of atherosclerosis, and acute refractory spasm can be lethal [1, 2]. The mechanism of graft spasm is not clearly understood, and the most effective treatment is still controversial [3]. Pretreatment with a vasodilator drug of the graft ex vivo or intraluminal injection before implantation is a topical method used for spasm prophylaxis [4]. A variety of vasodilator drugs with vasodilator properties have been investigated to prevent graft spasm [4–8]. Single direct application of topical vasodilators on the graft in clinical practice raises concerns about the efficacy, duration, and safety of the vasodilator drug. Papaverine is a commonly used agent to this aim. Limited efficacy, duration of action, and safety concerns are papaverine’s main disadvantages [9]. The search for an efficient, long-lasting, and safe drug and a delivery method continues.
Botulinum toxin is an exotoxin released by the organism Clostridium botulinum. It rapidly and irreversibly binds to the presynaptic neuron and acts by preventing the release of acetylcholine from the presynaptic terminal. It takes up to 6 months for the neuromuscular function to return completely [10]. Botulinum toxin has 7 serological types: A through G [11]. Botulinum toxin serotype A (BTX-A) is commercially available for clinical use. It has found broad use in the treatment of various conditions involving striated and smooth muscles, including ophthalmic disorders, movement disorders, muscle spasticity, pain syndromes, and overactivity of smooth muscles [11]. Recent animal studies show that botulinum toxin is effective for the prevention of arterial graft spasm in rat abdominal aorta [12]. Currently, there is no information on the effects of BTX-A on the human saphenous vein and internal mammary artery grafts that are used in clinical practice.
This in vitro study was designed to assess the vasoactive effects and time-dependent changes of BTX-A and papaverine pretreatment on vasospasm of human SV and IMA grafts.
Material and methods
The study was approved by the local Institutional Ethics Committee (project number: 2016494). Written consent of the patients undergoing myocardial revascularization was obtained for the use of otherwise discarded artery and vein grafts. SV segments were obtained from 42 patients: 34 males and 8 females, with a mean age 60.7 years (range: 34–81), and the IMA segments were from 48 patients: 42 males and 6 females, with a mean age of 64.2 years (range: 32–84). Vessel segments from patients with a history of diabetes were not included. Preoperative cardiac treatment, such as calcium channel blockers, ACE inhibitors, and nitrates, were discontinued 24 h prior to surgery. SV and IMA segments were placed in cold Krebs-Henseleit solution (KHS) and immediately transferred to the laboratory.
Tissue samples
After careful removal of the surrounding tissue, artery and vein segments were cut into rings of 2 to 3 mm width and suspended in a 10 ml organ bath containing KHS at 37°C and continuously bubbled with 95% O2 and 5% CO2 gas mixture similarly to the previously described method [12]. Two or three rings were obtained from each vessel segment. Eight rings from different patients were randomly allocated to different groups with sequentially numbered samples to eliminate the influence of preoperative drug therapy. Each experiment was conducted on a separate ring. Each ring was exposed to only one constricting agent. Isometric contraction responses were recorded using a transducer (BIOPAC MP36, CA, USA) and a digitized data acquisition system (Commat, Ankara, Turkey).
At the beginning of each experiment, the rings were stretched to an initial resting tension of 2 g and allowed to equilibrate for 60 min in the KHS, which was changed every 15 min before the administration of vasoactive drugs as described. After this equilibrium period, the following protocols were conducted.
Experimental protocols
A summary of experimental protocols for BTX-A and papaverine groups and sample sizes for each protocol are displayed in a flow chart (Figure 1).
Figure 1
Flow chart of experimental procedures
SV – saphenous vein, IMA – internal mammary artery, KCL – potassium chloride, 5-HT – 5 hydroxytryptamine, ET-1 – endothelin-1, n – number of strips.
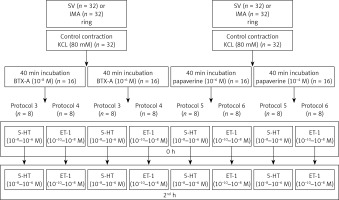
To study the vasodilator effects of BTX-A and papaverine, 5-hydroxytryptamine (5-HT) and endothelin-1 (ET-1) were used as vasoconstrictor agents in the study groups.
Protocol 1: Responses to BTX-A on the basic tone of SV and IMA; the SV and IMA ring at resting tension were treated with 10–8 M and 10–6 M BTX-A, and responses were recorded (Figure 1, Protocol 1).
Protocol 2: Control contractions for 5-HT and ET-1; to test the viability of the vessels and to obtain a standard control contraction for the following experiments, contraction responses with 80 mM KCl were obtained and recorded for each group of rings at resting tension, and the rings were washed and allowed to equilibrate for 1 h. After equilibration, cumulative 5-HT (10–8–10–6 M) was added to the organ bath, and dose-response curves were obtained. This procedure with 5-HT was repeated 2 h later on the same rings. In another group of rings the same procedures were employed with cumulative ET-1 (10–10–10–8 M) (Figure 1, Protocol 2). These dose-response curves were considered as control contractions of inhibitory agents in each group of rings for protocols 3, 4, 5, and 6.
Protocols 3 and 4: The effects of incubation with BTX-A on contractions of 5-HT and ET-1; in different vessels, control contraction responses with 80 mM KCl were obtained and recorded for each SV and IMA ring at resting tension, and the rings were washed and allowed to equilibrate for 1 h. Two different concentrations of BTX-A (10–6 or 10–8 M) were added to the organ bath in 2 different groups, and the rings were incubated for 40 min. Then the rings were washed. After equilibration, cumulative 5-HT (10–8–10–6 M) (Figure 1, Protocol 3) or ET-1 (10–10–10–8 M) (Figure 1, Protocol 4) were added to the organ bath, and dose-response curves were obtained (0 h). The protocols were repeated 2 h later in the same rings (2nd h).
Protocols 5 and 6: The effects of incubation with papaverine on contractions of 5-HT and ET-1.
Protocols 3 and 4 were repeated on a different group of rings with 2 different concentrations of papaverine (10–6 and 10–4 M) instead of BTX-A (Figure 1, Protocol 5 and 6).
Histology
A group of untreated SV and IMA rings (n = 8) together with rings incubated with each concentration of BTX-A (10–8 M and 10–6 M) (n = 8) and papaverine (10–4 and 10–6 M) (n = 8) were evaluated by histological examination for tissue integrity. All samples were sent to the pathology laboratory in a 10% formaldehyde solution. After routine tissue preparation and blocking procedures, 5-μm-thick slices were obtained. They were stained with haematoxylin and eosin, and all of the vascular layers (intima, media, and adventitia) were examined under a light microscope for fibrosis, necrosis, and any other pathological changes.
Statistical analysis
SPSS for Windows (version 17.0) (SPSS Inc., Chicago, IL, USA) was used for statistical analysis. Dose-response results for each concentration of the constrictor agent (5-HT and ET-1) was evaluated as the percentage of control contraction responses elicited by KCl. Data are presented as mean ± SD for continuous variables. The Shapiro-Wilk test was used to assess normal distribution of numeric values. The data showed normal distribution. Student’s t-test was used to compare the independent groups with control results. Student’s paired t-test was used to compare the 0 and 2nd h results of dependent groups. P < 0.05 was considered significant.
Drugs
The following drugs were used: 5-HT, papaverine, and ET-1 were purchased from Sigma Aldrich, (St. Louis, MO, USA) and BTX-A from Allergan Pharmaceuticals plc, (Dublin, Ireland). KH solution was prepared in the laboratory with compositions as follows (in mM): NaCl 118.3, KCl 4.69, KH2PO4 1.18, CaCl2 1.25, MgSO4 1.17, NaHCO3 25.0, glucose 11.1.; pH: 7.4.
Results
The results of the control and study groups are presented in Table I.
Table I
Effect of incubation with BTX-A and papaverine on 5-HT- and ET-1-induced contractions. Results are presented as percentage of contractions induced by KCL
Constrictor concentration | Incubation agent | SV | IMA | |||
---|---|---|---|---|---|---|
0 h | 2nd h | 0 h | 2nd h | |||
5-HT | 10–8 M | Control | 101.63 ±14.99 | 93.29 ±4.53 | ||
BTX-A (10–8 M) | 95.71 ±20.41 | 81.29 ±15.31*Ψ | 79.14 ±10.99* | 77.43 ±10.2* | ||
BTX-A (10–6 M) | 76.43 ±12.12* | 57.14 ±10.10*Ψ | 70.43 ±10.19* | 64.57 ±11.74* | ||
Pap (10–6 M) | 74.57 ±18.93* | 83.57 ±12.68* | 84.86 ±4.37* | 89.57 ±4.50Ψ | ||
Pap (10–4 M) | 21.14 ±0.11* | 49.14 ±8.27*Ψ | 61.75 ±6.27* | 74.38 ±8.45*Ψ | ||
10–6 M | Control | 139.25 ±26.44 | 107.43 ±6.02 | |||
BTX-A (10–8 M) | 123.43 ±17.90 | 107.71 ±11.54*Ψ | 93.00 ±4.86* | 87.57 ±5.50* | ||
BTX-A (10–6 M) | 99.57 ±11.20* | 81.43 ±9.99*Ψ | 85.29 ±5.67* | 75.43 ±10.98*Ψ | ||
Pap (10–6 M) | 103.00 ±9.38* | 117.43 ±11.97 | 93.00 ±4.50* | 99.14 ±3.43*Ψ | ||
Pap (10–4 M) | 29.71 ±13.75* | 61.29 ±25.99*Ψ | 70.00 ±7.07* | 89.25 ±6.27*Ψ | ||
ET-1 | 10–10 M | Control | 48.25 ±13.81 | 82.86 ±4.18 | ||
BTX-A (10–8 M) | 34.25 ±14.11* | 29.50 ±10.02*Ψ | 70.63 ±9.73* | 66.88 ±11.15*Ψ | ||
BTX-A (10–6 M) | 21.75 ±5.31* | 18.38 ±3.02*Ψ | 56.00 ±6.71* | 55.13 ±6.19*Ψ | ||
Pap (10–6 M) | 43.29 ±9.75 | 53.57 ±7.04Ψ | 76.14 ±4.52* | 82.14 ±3.67Ψ | ||
Pap (10–4 M) | 34.43 ±6.21* | 44.43 ±8.14Ψ | 63.43 ±9.71* | 71.86 ±7.71*Ψ | ||
10–8 M | Control | 67.88 ±11.65 | 97.43 ±3.50 | |||
BTX-A (10–8 M) | 40.75 ±12.83* | 37.50 ±12.41*Ψ | 80.88 ±9.20* | 79.50 ±9.11* | ||
BTX-A (10–6 M) | 27.00 ±5.58* | 23.88 ±3.48*Ψ | 66.13 ±5.35* | 62.75 ±4.92*Ψ | ||
Pap (10–6 M) | 55.43 ±7.30* | 67.43 ±7.78Ψ | 82.86 ±3.53* | 90.00 ±4.39*Ψ | ||
Pap (10–4 M) | 39.43 ±4.75* | 50.86 ±5.46*Ψ | 69.29 ±7.06* | 79.57 ±3.56*Ψ |
BTX-A (10–8 M and 10–6 M) did not affect the resting tone of SV and IMA rings.
Control contraction responses of 5-HT (10–8–10–6 M) and ET-1 (10–10–10–8 M) were 101.63 ±14.99% – 139.25 ±26.44%, and 48.25 ±13.81% – 67.88 ±11.65%, respectively, for SV and 93.29 ±4.53% – 107.43 ±6.02% and 82.86 ±4.18% – 97.43 ±3.50%, respectively, for IMA.
Responses were concentration-dependent, repeatable, and did not show time-dependent changes.
Results of BTX-A on SV rings (Figure 2)
Incubation of SV rings with 10–8 M BTX-A did not influence the maximum constriction responses of 10–8 M 5-HT and 10–6 M 5-HT at 0 h compared to control but significantly inhibited the contractile response to both concentrations of 5-HT at the 2nd h (p < 0.05). However, the higher concentration of BTX-A (10–6 M) inhibited all contraction responses elicited by 10–8 M 5-HT and 10–6 M 5-HT, both at the 0 and 2nd h (p < 0.05). When the 0 and 2nd h were compared, the inhibition was significantly higher at the 2nd h with both concentrations of BTX-A (p < 0.05).
Figure 2
Effects of botulinum toxin A pretreatment on constriction elicited by 5-HT and ET-1 on saphenous vein rings. Results are expressed as percentage of KCl-induced control contraction (mean ± SD). *P < 0.05 vs. control and §p < 0.05 vs. 0 h
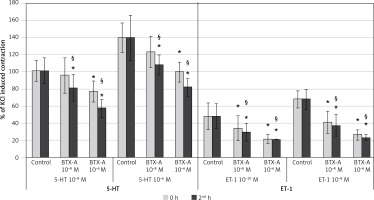
Incubation with both concentrations of BTX-A resulted in a significant inhibition in the maximum constriction responses elicited by both 10–10 M of ET-1 and 10–8 M of ET-1 at both time points (0 and 2nd h) (p < 0.05). Moreover, it was found that the inhibitory effect of BTX-A increased significantly at the 2nd h for all ET-1 experiment groups (p < 0.05).
Results of papaverine on SV rings (Figure 3)
When evaluating the effect of incubation with the lower concentration of papaverine (10–6 M) on 10–8 M 5-HT, it was seen that papaverine significantly inhibited the contractile response at both the 0 and 2nd h (p < 0.05). The degree of inhibition was similar between the 0 and 2nd h. Also, papaverine (10–6 M) significantly inhibited the contractile response to 10–6 M 5-HT at 0 h (p < 0.05), whereas this inhibition resolved entirely at the 2nd h. Incubation with the higher concentration of papaverine (10–4 M) significantly inhibited the maximum constriction responses of both 10–8 M 5-HT and 10–6 M 5-HT at both the 0 and 2nd h (p < 0.05). The inhibition at the 2nd h significantly decreased compared to the 0 h with both concentrations of 5-HT.
Figure 3
Effects of papaverine pretreatment on constriction elicited by 5-HT and ET-1 on saphenous vein rings. Pap: papaverine, results are expressed as percentage of KCl-induced control contraction (mean ± SD). *p < 0.05 vs. control and #p < 0.05 vs. 0 h
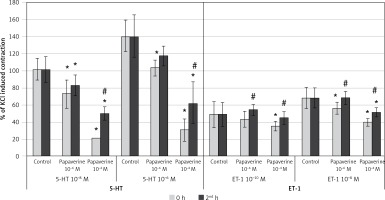
The lower concentration of papaverine (10–6 M) did not inhibit the contractile response to 10–10 M of ET-1 at both the 0 and 2nd h. The same concentration of papaverine significantly inhibited the contractile response to 10–8 M of ET-1 at the 0 h (p < 0.05) but not at the 2nd h. Higher concentration of papaverine (10–4 M) significantly inhibited the contractile response to 10–10 M of ET-1 at the 0 h (p < 0.05), whereas this inhibition discontinued at the 2nd h. The same concentration of papaverine significantly inhibited the contractile response to the higher concentration of ET-1 (10–8 M) at both the 0 and 2nd h (p < 0.05). The decrease in inhibition was significant at the 2nd h (p < 0.05).
Results of BTX-A on IMA rings (Figure 4)
Incubation of IMA rings with both 10–8 M and 10–6 M BTX-A significantly inhibited the contractile response elicited by both concentrations of 5-HT (10–8 and 10–6 M) at the 0 and 2nd h (p < 0.05). With the higher concentration of BTX-A (10–6 M), only the maximum contractile response to 10–6 M 5-HT decreased significantly at the 2nd h compared to the 0 h (p < 0.05). The inhibition at the 2nd h was similar to the 0 h in the other 3 groups.
Figure 4
Effects of botulinum toxin A pretreatment on constriction elicited by 5-HT and ET-1 on internal mammary artery rings. Results are expressed as percentage of KCl-induced control contraction (mean ± SD). *P < 0.05 vs. control and §p < 0.05 vs. 0 h
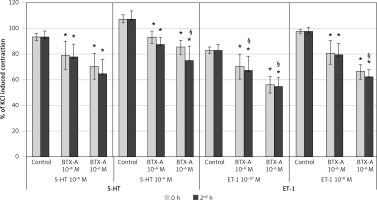
Both 10–8 M and 10–6 M BTX-A significantly inhibited the contractile response to both concentrations of ET-1 (10–10 and 10–8 M) at the 0 and 2nd h (p < 0.05). When the 0 and 2nd h were compared, the inhibition was significantly higher at the 2nd h (p < 0.05) in all groups except for the lower concentration of BTX-A (10–8 M) and the higher concentration of ET-1 (10–8 M).
Results of papaverine on IMA rings (Figure 5)
Incubation of IMA rings with papaverine (10–6 M) significantly inhibited the contractile response to 10–6 M 5-HT both at the 0 and 2nd h (p < 0.05). When the 0 and 2nd h were compared, this inhibition was significantly greater at the 0 h (p < 0.05). Compared to the control contraction, the inhibition of maximum constriction response to 10–8 M 5-HT after incubation with 10–6 M papaverine was significant at the 0 h (p < 0.05) whereas the inhibition was considered as non-significant at the 2nd h. On the other hand, incubation with the higher concentration of papaverine (10–4 M) significantly inhibited the contractile response to both concentrations of 5-HT at the 0 and 2nd h (p < 0.05). In these groups, a significant decrease in the inhibition of 5-HT-induced constriction was observed at the 2nd h in both concentrations (p < 0.05).
Figure 5
Effects of papaverine pretreatment on constriction elicited by 5-HT and ET-1 on internal mammary artery rings. Pap: papaverine, results are expressed as percentage of KCl-induced control contraction (mean ± SD). *P < 0.05 vs. control and #p < 0.05 vs. 0 h
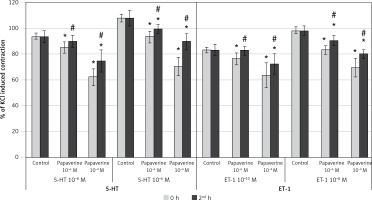
10–6 M papaverine significantly inhibited the contractile response of IMA rings to 10–10 M of ET-1 at the 0 h (p < 0.05) whereas the inhibition discontinued at the 2nd h. The same concentration of papaverine significantly inhibited the contractile response to 10–8 M of ET-1 both at the 0 and 2nd h (p < 0.05). When the 0 and 2nd h were compared, the inhibition significantly decreased in time (p < 0.05). Incubation with the higher concentration of papaverine (10–4 M) significantly inhibited the contractile response to both concentrations of ET-1 (10–10 M and 10–8 M) both at the 0 h and the 2nd h (p < 0.05). The inhibitory effect on contractions obtained by both concentrations of ET-1 showed a significant change in time and decreased at the 2nd h (p < 0.05).
Histological evaluation of vessels treated with BTX-A and papaverine under light microscopy showed similar findings to untreated vessels. All of the vascular layers (intima, media, and adventitia) retained their integrity. None of the samples displayed signs of fibrosis, inflammation, or necrosis (Figure 6).
Figure 6
Cross sectional slices of human graft vessels stained with haematoxylin and eosin (H + E). Under light microscope, no histologic changes were present between untreated internal mammary artery (IMA) segments (A) and IMA segments pretreated with 10–4 M of papaverine (B), 10–6 M of papaverine (C), 10–6 M of BTX-A (D), and 10–8 M of BTX-A (E). Similarly, no histological changes were present between untreated saphenous vein (SV) segments (F) and SV segments pretreated with 10–4 M of papaverine (G), 10–6 M of papaverine (H), 10–6 M of BTX-A (I), and 10–8 M of BTX-A (J)
BTX-A – botulinum toxin A.
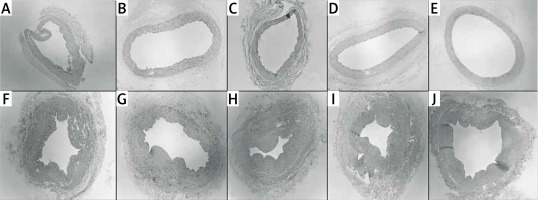
Discussion
In this study, we investigated the vasodilatory and histomorphological effects of BTX-A and papaverine on precontracted human SV and IMA grafts. Our results suggest the following: 1) incubation with BTX-A and papaverine relaxes both SV and IMA rings contracted with 5-HT and ET-1; 2) the duration of the relaxant effect of BTX-A is longer than that of papaverine; and 3) no apparent histomorphological changes were observed in the grafts under light microscopy with the present concentrations of BTX-A and papaverine.
Vasospasm of the conduits following CABG surgery may lead to a low-flow syndrome, which may result in cardiac ischaemia and infarction. Arteries used as grafts are more susceptible to spasm due to substantial muscle layers. Venous graft spasms are less commonly reported and are usually observed during the initial 48 h after surgery [13]. The mechanism of graft vasospasm is believed to be associated with the balance of vasodilator and vasoconstrictor influences as well as mechanical and physical stimulation and oxidative stress [3, 14].
For this study, we used 5-HT and ET-1 to induce vasoconstriction in vessel rings. 5-HT is a well-known neurotransmitter that promotes platelet aggregation and is contained in platelets in high amounts. Highest peripheral levels of 5-HT are found in the cardiovascular system. The vasoconstrictive action of 5-HT is mediated through 5-HT2 serotonergic receptors, and in arterial smooth muscle cells, 5-HT also amplifies the release and activities of other vasoconstrictors such as histamine, angiotensin II, prostaglandin F2α, and noradrenaline [15]. ET-1 is a potent constrictor of human vessels [16]. There are 2 basic types of ET-1 receptor: ETA and ETB. Endothelial cells express ETA, which increases NO production, whereas smooth muscle cells express both receptors, which results in prolonged and long-lasting vasoconstriction [17]. Patients with cardiovascular disease have elevated serum ET-1, which enhances vasoconstriction. Additionally, sub-pressor concentrations of ET-1 can also potentiate vasoconstrictor responses of IMA, and coronary artery rings to adrenaline, noradrenaline, and serotonin [18]. In the present study, compared to ET-1, 5- HT resulted in more powerful vasoconstriction in SV grafts. In IMA grafts both constrictors caused similar vasoconstriction. Variations in the response of veins and arteries to vasoconstrictors are considered to be due to biological heterogeneity of different vessels.
Topical administration of various agents with vasodilator properties have been investigated to prevent graft spasm after CABG surgery. An often-used method is to incubate the clamped graft during surgery by intra-luminal administration of a potent vasodilator agent after harvest [5–8, 19]. We selected papaverine as one of the study drugs because it is one of the most popular agents to this aim [4–7, 19]. Papaverine acts by inhibiting phosphodiesterase in smooth muscle cells, which leads to an increase in intracellular cyclic adenosine monophosphate (cAMP) and cyclic guanosine 3,5-monophosphate (cGMP), resulting in smooth muscle relaxation [20]. It regulates the intracellular calcium-mediated/myosin light chain phosphorylation pathway as well as the actin regulatory protein-mediated actin depolymerization pathway, both of which are involved in the relaxation of smooth muscle tissue [21]. The vasodilator action of papaverine is potent but of relatively short duration. The plasma half-life of papaverine is relatively short [6, 7].
In the present study, in SV, pre-treatment with both concentrations of papaverine caused an immediate inhibition in the 5-HT-induced contractions, which significantly decreased at the 2nd h. Low-dose papaverine was especially inadequate in inhibiting lower concentrations of ET-1-induced contractions, and the inhibition seen with high-dose papaverine decreased or discontinued at the second hour. Furthermore, papaverine was initially more effective in inhibiting the constriction of both 5-HT and ET-1 in IMA, but the inhibitory effect decreased or entirely resolved at the second hour. Hocking et al. similarly showed that in human SV papaverine-induced inhibition of constriction elicited by norepinephrine was reversible in a time-dependent manner [21]. In a recent study on human RA grafts, we found that the relaxing effects of BTX-A and papaverine change according to the contracting agent [22]. In the RA, the inhibitory effect of papaverine was stronger but shorter than that of BTX-A on 5-HT elicited contractions. The inhibitory effects of BTX-A and papaverine were similar on ET-1-mediated contractions initially; however, BTX-A showed stronger inhibition than papaverine at the 2nd h. Likewise, Mussa et al. found that in human RA, when compared with topical phenoxybenzamine and verapamil/nitroglycerin solutions, papaverine resulted in the shortest duration of action, with limited efficacy against relevant vasoconstrictors. They concluded that the limited duration of action against both receptor-mediated and receptor-independent vasoconstriction is insufficient to recommend the use of papaverine as an effective topical antispasmodic preparation [7]. Yoshizaki et al. reviewed angiographic results among 116 patients receiving CABG, who had RA grafts and were treated with either verapamil-nitroglycerin or papaverine. The results showed that papaverine was significantly associated with RA graft occlusion [9]. Dipp et al. compared the efficacy of papaverine and phenoxybenzamine to prevent radial artery spasm and their ability to preserve endothelial function. While papaverine prevented spasm for up to a maximum of 30 min, phenoxybenzamine prevented spasm for at least 6 h. Papaverine caused damage in the endothelium of 70% of vessels, whereas phenoxybenzamine showed no evidence of endothelial damage in any arterial segments [23]. There is also previous work demonstrating that incubation with papaverine preserves endothelial integrity in RA segments [24]. In the present study, to avoid vascular damage we selected a concentration range taking into account the earlier studies that were capable of preventing vasoconstriction while maintaining endothelial function [7, 21]. In our present results, pretreatment with the higher concentration of papaverine did not cause any damage to the vessel structure. The different results on vessel damage may be due to the concentration and pH of the study solutions as well as the different vessels used in the studies.
BTX-A is the main serotype of botulinum toxin. Since it was introduced for clinical use in 1977, it has proven to be effective and safe in the treatment of various disorders [25]. The FDA approved BTX-A for use including moderate to severe glabellar lines, hyperhidrosis, blepharospasm, ocular strabismus, cervical dystonia, and torticollis [10, 11, 25]. When injected locally BTX-A has a limited diffusion and weakness of distant muscles, or generalized weakness is very rare [10]. BTX-A rapidly binds and affects the injected muscle. The unbound toxin is washed away and diluted to a decreased concentration in larger and larger volumes of extracellular fluids, incapable of binding to the presynaptic membrane [11].
In vitro studies have demonstrated that BTX-A is effective in inhibiting smooth muscle spasms [26]. As a result of this long-lasting inhibition on smooth muscle, research has focused on disorders of vascular origin. Hayashi et al. documented a partial increase in blood flow in the femoral artery in rats [27]. Fathi et al. showed that pre-treatment with BTX-A was associated with a significantly larger arterial and venous diameter before microanastomosis. Moreover, BTX-A was also associated with a lower rate of arterial and venous thrombosis after microanastomosis [28]. BTX-A has received increasing attention as a means of relieving symptoms of vasospasm in Raynaud’s phenomenon [29, 30]. BTX-A inhibits muscle contraction by preventing the release of acetylcholine from the nerve terminal by blocking the transport of vesicles that contain acetylcholine. Thus, the neurotransmitter is not released into the synaptic cleft, resulting in long-term paralysis [31]. Additionally, it prevents sympathetic vasoconstriction of the vascular smooth muscle by blocking the transmission of the norepinephrine vesicle, and it blocks recruitment of the specific α2-adrenoceptor, which decreases the activity of chronically upregulated C-fibre nociceptors [32]. BTX-A interacts with the Rho/Rho kinase. BTX-A inactivates Rho kinase and inhibits smooth muscle cell constriction directly through interference with the Ca2+ sensitivity of vascular smooth muscle cells and the NO system [33]. BTX-A duration of action differs from papaverine. BTX-A injections can exert prolonged effects for up to 6 months [10, 11, 34].
Our results are consistent with the findings of Murakami et al. [12], who, in a similar experimental model, compared papaverine with botulinum toxin C (BTX-C) for prevention of arterial graft spasm in rat abdominal aorta in the presence of KCl and noradrenaline. They demonstrated that all concentrations of BTX-C could prevent arterial spasm in isolated rat aorta rings and had a longer preventive effect than papaverine with no toxic effect on the arterial structure of the rat aorta. In the present study pretreatment with BTX-A resulted in immediate inhibition of vasoconstriction both in SV and IMA, which persisted and even increased at the 2nd h in SV and in IMA depending on the concentration of the solution, the constrictor agent, and the structure of the graft. No damage on the grafts was observed with both concentrations of BTX-A.
There are several limitations to this in vitro study. Graft vasospasm is multifactorial; therefore, it is not possible to study in vitro all factors that might cause vasoconstriction of homografts. Our results may change in vivo with the complex counterbalancing of vasodilators and vasoconstrictors. The present study was conducted on remnant grafts obtained from a limited number of patients. We only studied 2 potent vasoconstrictors at 2 clinically relevant concentrations. The duration was limited to 2 h. In vivo models may provide more precise information on the potency and duration of BTX-A and papaverine as topical vasodilators.
In conclusion, this study demonstrates that in human SV and IMA grafts, pretreatment with both BTX-A and papaverine are safe and have a potent inhibitory effect depending on the vessel and vasoconstrictor agent. The long-lasting vasodilatory effect of BTX-A on vascular smooth muscle may provide promising results for the prevention venous and arterial graft spasm. Further in vitro and in vivo investigations are warranted.