Introduction
Thymoquinone (2-isopropyl-5-methyl-1,4-benzoquinone), generally abbreviated TQ, is one of the phytochemical ingredients in the seeds and volatile oil extract of Nigella sativa (known as black seed or black cumin) [1]. Thymoquinone is reportedly a safe nutrient, during oral intake to experimental animals [2]. Thymoquinone has been documented to elicit many effects [3], including immunomodulatory, anticancer, antidiabetic, antioxidant, anti-infertility and anti-inflammatory activities, as well as protection of the liver, heart and nervous systems. Diabetes mellitus (DM) as one of the metabolic disorders, is characterized by hyperglycemia, probably due to pancreatic dysfunction, insulin resistance, or both [4]. Clinically, the number of patients with DM has gradually increased worldwide, and the prevalence of DM has been predicted to reach 439 million people by 2030 [5]. Recently, incretin therapy has been widely used in DM treatment [6] and two strategies have been applied in clinics around the world: application of analogs of glucagon-like peptide-1 (GLP-1) and/or inhibitors of dipeptidyl peptidase-IV (DPP-4) that degrade GLP-1 rapidly to endogenous levels. However, the currently available drugs can have significant side effects and can also be quite expensive [7].
Thymoquinone is known to reduce hepatic glucose production in diabetic animals [8]. In clinics, black seed (black cumin) produced hypoglycemic and hypolipidemic effects in patients suffering from diabetes and other metabolic syndromes [9]. Thymoquinone has protective effects on the kidney and liver against target organ damage [10]. Therefore, development of TQ as a useful adjunct for DM appears clinically beneficial.
In clinics, metformin as a widely used antidiabetic drug has also been indicated to enhance plasma GLP-1 levels in healthy individuals and persons with type-2 diabetes [11, 12]. Further studies have indicated that metformin may activate the imidazoline receptors [13]. The imidazoline receptor activated by the natural product also reportedly results in attenuation of hyperglycemia in diabetic animals [14]. The chemical structure of TQ is similar to imidazolines. It appears possible that TQ may activate imidazoline receptors to enhance GLP-1 secretion. Therefore, it is of special interest to clarify the changes in GLP-1 levels in the face of TQ-induced hypoglycemic effects after the activation of imidazoline receptors.
Material and methods
Materials
Thymoquinone (purity > 98%) purchased from the Sigma-Aldrich Chemical Co. (St. Louis, MO, USA) and BU-224 purchased from Tocris Bioscience (Fisher Scientific, Leicestershire, UK) were dissolved in normal saline. Additionally, the present study also purchased NISCH cDNA ORF clone (Origene, Rockville, MD, USA), anti-β-actin antibody (Sigma-Aldrich, St. Louis, MO, USA), and anti-NISCH antibody (Abcam, Cambridge, MA, USA).
Cell cultures
Human NCI-H716 cells (BCRC No. CCL-251) and CHO-K1 cells (BCRC No. CCL-61) were obtained from the Culture Collection and Research Center (BCRC) of the Food Industry Institute (Hsin-Chiu City, Taiwan). Cells subcultured every 3 to 4 days in medium containing 10% fetal bovine serum (FBS) were applied between passages 18 and 25 in the present study.
Transfection of NISCH in CHO-K1 cells
Nischarin is generally used as a functional imidazoline receptor because it is a mouse homolog of the human imidazoline receptor antisera-selective (IRAS) protein [15]. According to a previous report [16], an expression vector containing human NISCH cDNA (NISCH) (Myc-DDK-tagged human nischarin, NM_007184; Origene Technologies, Inc., Rockville, MD, USA) was stably transfected into CHO-K1 cells using the TurboFect transfection reagent (Thermo Fisher Scientific, Rockford, IL, USA). After 24 h of transfection, expression in cells was confirmed using Western blotting analysis (described below) and the cells were used to incubate with TQ for further experiments.
Western blotting analysis
The cells were lysed to extract the protein and thirty micrograms of the cell lysates were separated using 10% sodium dodecylsulfate polyacrylamide gel electrophoresis. Then, they were transferred to a polyvinyl difluoride membrane. After blocking with 10% skim milk for 1 h, the blots were developed with a primary antibody specific for NISCH. The secondary antibodies were then incubated with membranes for an additional 3 h. The mixed complexes were determined using an ECL kit (Thermo Fisher Scientific). Using a marker to verify specificity, immunoblots indicating NISCH (37 kDa) and β-actin (43 kDa) were obtained.
Estimation of intracellular calcium level
Intracellular calcium levels have been widely determined using the fluorescent probe fura-2 [14]. According to our previous report [17], NISCH-CHO-K1 cells were maintained in physiological saline solution (PSS). Fura-2 (5 mM) was added to 1 ml of the cell suspension (1 × 106 cells) to incubate at 37°C for 30 min in the dark. After addition of a testing substance of vehicle, the fluorescence was continuously recorded using a fluorescence spectrofluorometer (Hitachi F-2000). Values of [Ca2+]i were detected and the background in unloaded cells was subtracted from all measurements.
Silencing of imidazoline receptors in GLP-1 secreting cells
Following a previous report [18], a validated small interfering RNA (siRNA) targeting human nischarin (NISCH) was purchased from a commercial source (GE Healthcare Dharmacon, Inc. Lafayette, CO, USA). Pooled non-targeting siRNA was used as a negative control to distinguish the silencing from nonspecific effects. Lipofectamine 2000 (Thermo Fisher Scientific, Pittsburgh, PA, USA) was applied to transfect the siRNA into NCI-H716 cells. After 24 h of transfection, Western blotting analysis was then used to evaluate the success of this silencing before assays.
Evaluation of GLP-1 secretion in vitro
NCI-H716 cells were seeded in 24-well plates (5 × 105 cells per well) to incubate in assay medium (DMEM 1 g/l glucose supplemented with 1 mmol/l pyruvate, 1% PenStrep, 0.1 mmol/l, DPP-IV inhibitor) containing desired concentration of TQ at 37°C for 1 h. Supernatants were collected and centrifuged (1,500 g, 5 min) to remove floating cells and debris for GLP-1 measurement. The concentrations of GLP-1 in the supernatants were then measured using an ELISA kit (Millipore, Burlington, MS, USA).
Animals
Male Sprague-Dawley (SD) rats, weighing 250 to 280 g, were supplied from the National Laboratory Animal Center (Taipei, Taiwan). Before the experiments, all protocols were approved by the Institutional Animal Ethics Committee (105051901) in Chi-Mei Medical Center. All experiments conformed to the Guide for the Care and Use of Laboratory Animals, as well as the guidelines of the Animal Welfare Act. Animals under sodium pentobarbital (35 mg/kg, i.p.) anesthesia were used in the present study to reduce their suffering.
Induction of diabetic rats
To induce the model of type 1-like diabetes, overnight fasted rats were used to receive an intravenous injection of streptozotocin (STZ) at 65 mg/kg. Success of the model was identified once they showed hyperglycemia, the plasma glucose level not less than 300 mg/dl measured from an automatic analyzer, in addition to polyuria and other diabetic features at 1 week later. The plasma insulin levels determined by a rat insulin ELISA kit (Mercodia, Uppsala, Sweden) were also extremely lowered in these rats. Then, experiments were started 2 weeks after the preparation of the diabetic model.
Determination of plasma GLP-1 levels in vivo
The plasma GLP-1 levels were estimated after the treatment with TQ at the desired dose. The treated fasting rats were received 2.5 g/kg of glucose by gavage as described previously [19]. Under anesthesia with sodium pentobarbital (35 mg/kg, i.p.), at 30 min following glucose load, blood samples (about 300 ml) collected from the femoral artery were kept in Eppendorf tubes containing EDTA and DPP-IV inhibitor (10 μl; Millipore). Blood samples were centrifuged at 1500 g for 10 min and the GLP-1 levels in plasma were then estimated using an ELISA kit (Millipore).
Changes of plasma glucose in diabetic rats that received TQ treatment
Diabetic rats received sitagliptin (10 mg/kg) daily through oral gavage to inhibit DPP-4 or the same volume of vehicle for 2 weeks. Then, TQ at the indicated dose was administered by intraperitoneal injection (i.p.) in overnight-fasted diabetic rats with or without pretreatment with sitagliptin. Additionally, varying doses of exendin 9-39 (Ex9-39; Sigma-Aldrich) were pretreated 30 min before the injection of TQ in diabetic rats with or without pretreatment with sitagliptin. Under anesthesia, blood samples were collected at 1 h after TQ administration. Then, plasma glucose levels were determined as above.
Statistical analysis
Data shown are the mean ± SEM from the sample number (n) in each group. Statistical analysis regarding the one-way analysis of variance (ANOVA), followed by Tukey’s post hoc comparison, was performed with the software SPSS 21. A p-value of 0.05 or less was defined as significant.
Results
Direct effect of TQ on imidazoline receptors in cells transfecting NISCH
The exogenous NISCH gene transfected into CHO-K1 cells following our previous method [17] was confirmed using Western blot and it is indicated in the upper section of Figure 1. The imidazoline receptor (I-R) expressed in NISCH-CHO-K1 cells is proven to be functional [17].
Figure 1
Direct effect of thymoquinone (TQ) on imidazoline receptors (I-Rs) in vitro. Western blotting analysis confirmed the success of I-R gene transfection in CHO-K1 cells. Dose-dependent elevations in cellular calcium concentrations induced by TQ in I-R-transfected CHO-K1 cells (NISCH-CHO-K1 Cells) were observed compared with cells transfected with empty vector (CHO-K1 Cells). Values (means ± SEM) were obtained from 8 independent experiments
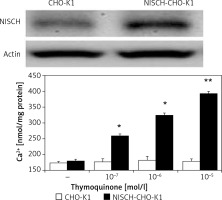
Then, the possible effects of TQ on I-Rs were investigated. In NISCH-CHO-K1 cells incubated with TQ, the intracellular calcium concentration was markedly elevated in a dose-dependent manner (Figure 1). However, similar changes induced by TQ treatment were not obtained in the original CHO-K1 cells that were not transfected with an exogenous I-R gene (Figure 1). Therefore, a direct effect of TQ on I-Rs could be determined.
TQ enhances GLP-1 secretion via imidazoline receptors in intestinal cells
Intestinal NCI-H716 cells have been widely used to evaluate GLP-1 secretion [20]. TQ enhanced GLP-1 secretion in NCI-H716 cells, as shown in Figure 2, and this effect of TQ was inhibited after silencing of the I-R gene, which dominated in NCI-H716 cells. Activation of I-Rs by TQ therefore enhanced GLP-1 secretion. Interestingly, this effect of TQ was also blocked by BU-224 pre-treatment in a dose-dependent manner (Figure 2).
Figure 2
Thymoquinone (TQ) treatment stimulated GLP-1 secretion in NCI-H716 cells. A – Western blots showing successful I-R silencing in NCI-H716 cells, as indicated in the upper panel. TQ treatment markedly induced GLP-1 secretion in NCI-H716 cells, as shown in scrambled RNA-treated controls. However, the effects of TQ were abolished by siRNA specific for I-Rs in NCI-H716 cells. B – TQ-induced GLP-1 secretion in NCI-H716 cells was blocked by BU-224 treatment. Each value (mean ± SEM) was obtained from 8 independent experiments
*P < 0.05 compared with the vehicle-treated group (1st column). #P < 0.05 compared with the scramble-treated, TQ-stimulated group.
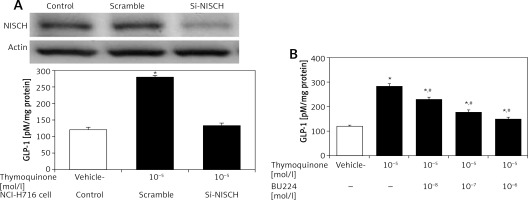
Elevation of plasma GLP-1 levels by TQ in diabetic rats
In the type 1-like diabetic rats, TQ treatment induced an increase in the plasma GLP-1 levels in a dose-dependent manner (Figure 3). Moreover, BU-224 pre-treatment also dose-dependently reversed the TQ-induced changes in these diabetic rats (Figure 3). However, similar treatment with BU-224 only at the most effective dose (1 mg/kg) did not influence the GLP-1 level in these diabetic rats compared with the vehicle-treated group (7.83 ±0.34 pg/ml vs. 7.24 ±0.65 pg/ml, p > 0.05, n = 8).
Figure 3
Effects of thymoquinone (TQ) on plasma GLP-1 levels in type 1-like diabetic rats. A – The plasma GLP-1 levels were reduced in diabetic rats (diabetes) as compared with the normal rats (first column) and administration of TQ at the indicated dose increased the plasma GLP-1 levels more markedly than in the vehicle-treated group (2nd column). B – BU-224 pretreatment inhibited the TQ-induced increase of plasma GLP-1 levels in diabetic rats. Diabetic rats were pretreated with BU-224 at the indicated dose for 30 min. Values are expressed as the mean ± SEM of 8 samples
*P < 0.05 compared with the vehicle-treated diabetic group. #P < 0.05 compared with the basal diabetic group without TQ treatment.
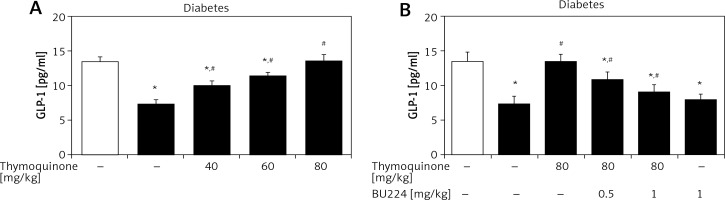
Functional identification of the effects of TQ on GLP-1 associated action in vivo
In type 1-like diabetic rats, TQ treatment may reduce the hyperglycemia in a dose-dependent manner (Figure 4). The plasma insulin levels in these diabetic rats (46.24 ±14.35 pmol/l, n = 8) were significantly different (p < 0.01) from those in normal rats (185.65 ±25.20 pmol/l, n = 8). TQ treatment at the most effective dose did not influence the plasma insulin levels in these diabetic rats (42.67 ±18.06 pmol/l, n = 8). Therefore, mediation of endogenous insulin in the effect of TQ seems negligible in the type-1 diabetic model. Additionally, hyperglycemia was reduced in these diabetic rats by sitagliptin treatment due to inhibition of DPP-4. The decrease in hyperglycemia by TQ was markedly potentiated by sitagliptin in these diabetic rats (Figure 4). Moreover, Ex9-39 treatment reversed the TQ-induced changes in these diabetic rats whether they received sitagliptin pre-treatment or not (Figure 4). However, Ex9-39 alone at the most effective dose did not influence the plasma glucose level in the diabetic rats compared with that in the vehicle-treated group (387.18 ±16.13 mg/dl vs. 394.53 ±17.68 mg/dl, n = 8, p > 0.05). Also, the same treatment with Ex9-39 did not modify the plasma glucose in diabetic rats treated with sitagliptin compared that in vehicle-treated rats receiving sitagliptin pre-treatment (342.53 ±16.44 mg/dl vs. 336.14 ±14.5 mg/dl, p > 0.05, n = 8).
Figure 4
Effects of thymoquinone (TQ) treatment on plasma glucose levels in type 1-like diabetic rats. A – The plasma glucose levels were measured 1 h after administration of TQ at the indicated dose in diabetic rats that received vehicle treatment or sitagliptin (10 mg/kg per day orally) for 14 days. B – Exendin 9-39 treatment inhibits the TQ-induced reduction in hyperglycemia in diabetic rats that received vehicle or sitagliptin for 14 days. Animals were pretreated with exendin 9-39 at the indicated dose for 30 min. The values are expressed as the mean ± SEM of 8 samples
*P < 0.05 compared with the vehicle-treated diabetic group (each white column at same condition). #P < 0.05 compared with the vehicle-treated diabetic rats (first white column). †P < 0.05 compared with the diabetic rats that received sitagliptin treatment (first black column).
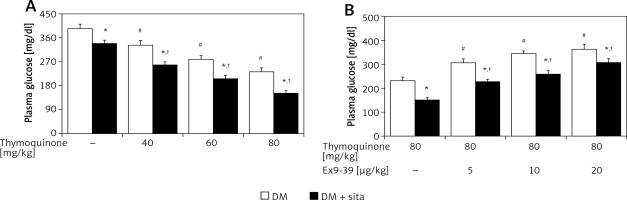
Discussion
Thymoquinone is the main active principle in the volatile oil of black seeds. TQ may reduce hepatic glucose production in diabetic hamsters [8] and abrogate the induction of STZ in a diabetic model [20]. The present study found that TQ might activate imidazoline receptors (I-Rs) to increase the plasma GLP-1 level in type-1 diabetic rats.
Initially, we characterized the direct effects of TQ on I-Rs using CHO-K1 cells that received a transient transfection of the I-R gene. Similar to the effect of another nutrient identified as an I-R agonist [14], TQ increased cellular calcium concentrations in cells that expressed the NISCH gene. Nischarin is known as a mouse homolog of human imidazoline receptor antisera-selective (IRAS) protein [21]. Human NISCH cDNA is widely used for transfection because it may serve as a functional imidazoline receptor [15]. The effectiveness of TQ disappeared in CHO-K1-cells without I-R expression. TQ may therefore be associated with the activation of I-Rs. We have shown, for the first time, the direct activation of I-Rs by TQ in vitro.
Then, the effects of TQ on I-Rs were further identified in GLP-1 secreting cell line NCI-H716 cells in vitro [22]. We used siRNA specific for NISCH to silence I-Rs in NCI-H716 cells and identified the decrease in I-R expression using Western blotting analysis. A dose-dependent elevation in GLP-1 secretion by TQ was observed in control-siRNA transfected NCI-H716 cells that showed the presence of I-Rs. Interestingly, the effectiveness of TQ was abolished in NCI-H716 cells receiving siRNA specific for NISCH. Moreover, GLP-1-releasing effects of TQ were also blocked by BU-224, an imidazoline I-2 receptor antagonist, in a dose-dependent manner [14, 16]. The activation of I-2 receptors by TQ can thus be considered. It is the first time the stimulatory effect of TQ on GLP-1 secretion via I-R activation in vitro has been indicated.
Plasma insulin levels in type-1 diabetic rats were extremely low, to be considered negligible, as described in a previous report [23]. High-dose STZ treatment induces direct toxic effects on β cells to reduce the endogenous insulin production [24]. The plasma insulin levels were not modified by TQ treatment in the present study. Therefore, the reduction of hyperglycemia by TQ through endogenous insulin appears unlikely. It is consistent with our previous findings [25]. Activation of the TGR5 receptor by another chemical such as glycyrrhizic acid may exhibit the same effects.
GLP-1 is involved in plasma glucose homeostasis [26]. The type-1 diabetic rats without the role of endogenous insulin [27] were applied to evaluate the in vivo effects of TQ. Similar to the effect on the cell model, TQ treatment increased the plasma GLP-1 levels in these diabetic rats in a dose-dependent manner. Interestingly, BU-224 pretreatment reversed the effect of TQ in these diabetic rats, indicating the mediation of I-2 receptor activation. Moreover, reduction of hyperglycemia by TQ treatment was potentiated in diabetic rats receiving sitagliptin at a dose sufficient to inhibit DPP-4 [28]. It is similar to that induced by activation of TGR5 [25]. Additionally, blockade of GLP-1 receptor using Ex9-39 [29] also diminished the effects of TQ on hyperglycemia in diabetic rats that received sitagliptin and those that did not. Therefore, GLP-1 is identified to be involved in the reduction of hyperglycemia by TQ. Moreover, these effects of TQ were also reversed by BU-224 pre-treatment at doses sufficient to block I-2 receptors. Therefore, TQ may activate I-Rs to enhance GLP-1 secretion for attenuation of hyperglycemia in type-1 diabetic rats. It shows a new potential for TQ-induced reduction of hyperglycemia.
Furthermore, GLP-1 has been reported to delay diabetes induction in animal models [30]. This is similar to a previous report that TQ can prevent STZ-induced diabetic model establishment [31]. Endogenous glucose production was directly inhibited by GLP-1 in humans [32]. Agonist(s) of GLP-1 were therefore suggested as adjunctive therapies for patients with type-1 diabetes (T1DM) [33]. Regarding the mechanism(s) underlying GLP-1-induced hypoglycemia in T1DM, an acute effect on gastric emptying and an effect on glucagon suppression were suggested [34]. Therefore, insulinotropic effects of GLP-1 through receptor activation and the insulin mimetic functions independent of receptors could be considered [35]. This is consistent with the extrapancreatic effects of GLP-1 [36]. However, a direct effect of TQ on glucose homeostasis (shall be concerned) for the reduction of hyperglycemia. Moreover, discovery of new agents as I-R agonists [17] would be a promising direction for diabetes treatment in the future.
In conclusion, all considered, we suggest that TQ treatment may enhance plasma GLP-1 levels via I-R activation in type-1 diabetic rats. Therefore, TQ could have exciting future clinical applications.