Introduction
Cardiovascular diseases (CVD) are a group of diseases such as ischemia, coronary heart diseases and hypertension which involve the heart and blood vessels. CVD is one of the main causes of deaths in diabetic patients. Studies reveal that microvascular and macrovascular injuries are the major contributing factors for increased incidence of CVD [1]. In addition to these vascular injuries, both clinical and preclinical evidence demonstrates the presence of diabetes-mediated cardiomyopathy, which generally shows changes in cardiac cells, i.e. cardiomyocytes. ‘Diabetic cardiomyopathy’ (DC) is characterized by impaired diastolic function along with progression of cardiomyocyte apoptosis, hypertrophy and myocardial fibrosis [2].
The mechanisms involved in diabetes-mediated cardiac damage are complex and multi-factorial. The increased burden of reactive oxygen species due to the hyperglycemic condition is the prime contributor to diabetes-mediated cardiac complications [3]. Cardiac tissues of diabetic patients are more prone to oxidative damage compared to those of non-diabetics; under normal physiological conditions the burden of reactive oxygen species is eliminated by the antioxidant defense of the body. It has been evidenced that both hyperglycemic and diabetic conditions contribute to impairing the endogenous antioxidant system [4]. Once the burden of reactive oxygen species exceeds the capability of the body’s antioxidant system, the excessive reactive species start damaging the integrity of DNA, alter protein function, damage membrane lipids, and cause necrosis and apoptosis [5]. NADPH oxidase has been identified as one of the key sources for generating myocardial reactive oxygen species under diabetic conditions [6]. It is responsible for reducing NADPH to oxygen, which leads to generation of superoxide anion. The continuous activation of NADPH oxidase may further lead to decrease of intracellular NADPH, causing impairment of the antioxidant system [7]. Confirming the important character of oxidative stress in the development of diabetes-mediated cardiac complications, there is rising importance of the therapies involving antioxidants.
Fucoxanthin (FXN) is one of the most abundant carotenoids obtained from seaweeds [8]. Due to the presence of special functional groups which include a 5,6-monoepoxide structure and an allenic bond, FXN shows a variety of pharmacological activities such as antioxidant activity by curbing oxidative stress and an anti-inflammatory effect [9]. FXN has also been reported to show anticancer, anti-angiogenic, anti-apoptotic, anti-proliferative [10], anti-obesity and very importantly anti-diabetic activity [11]. Though previous studies have shown that FXN is an anti-diabetic agent, none of the reports have investigated the potential role of FXN in the pathogenesis of diabetic cardiomyopathy.
In the present study, we evaluated the role of FXN in diabetic cardiomyopathy by treating primary neonatal rat cardiomyocytes pre-exposed to a high glucose environment. We also studied the potential pathways involved.
Material and methods
Reagents and antibodies
Fucoxanthin (FXN) was procured from Sigma-Aldrich (USA). Fetal bovine serum (FBS) was bought from Sigma-Aldrich (USA). Dulbecco’s modified eagle’s medium (DMEM) and collagenase type-2 were obtained from Thermo Fisher (USA). Cell Counting Kit 8 (WST-8/CCK8) (ab228554) was procured from Abcam (USA). Dorsomorphin dihydrochloride (DDH), an AMPK inhibitor, was procured from Santacruz Biotech (USA). For determining oxidative stress markers, Lactate dehydrogenase (LDH) and Malondialdehyde (MDA) kits from Abcam (USA), Catalase (CAT) and Glutathione (GSH) assay kits were obtained from Sigma-Aldrich (USA). Superoxide Dismutase (SOD) and ROS kits were supplied by Thermo Fisher (USA). The PI and Annexin V-FITC kit was procured from BestBio, China. For TUNEL assay the kit was obtained from Roche, Germany. The Rac1 Activation Assay Kit and NADPH activity assay kit were obtained from Abcam (USA). The antibodies used for the study were p22phox, p-Akt, Akt, p-AMPKα, p67phox, Bcl-2, p-GSK-3β, Bax, β-actin and GSK-3β, obtained from Cell Signaling Tech. (USA), whereas p47phox and gp91phox were supplied by Santa Cruz Biotech, (USA).
Animal studies
For the present study Sprague-Dawley neonatal rats aged 1 to 3 days were chosen. The animals were provided by the central animal house of Wuhan Children’s Hospital. All the animal studies adhered strictly to the draft Animal protection law of the People’s Republic of China (2009) for experimental animals. The animal experiments received approval from the animal ethical committee of Wuhan Children’s Hospital, China; the approval number was WCH0784-A
Isolation of cardiomyocytes from neonatal rats, culture and treatment
The cardiomyocytes were isolated from the neonatal rats as per the procedure described previously [12]. The isolated cardiomyocytes were cultured in controlled atmospheric conditions of 5% CO2 at 37°C in DMEM supplemented with FBS (10%), streptomycin (100 mg/ml) and penicillin (100 U/ml). About 1 × 106 cells were used for every experiment. The cardiomyocytes were then exposed to a variety of glucose containing medium ranging from 5 to 50 mM/l of D-glucose for 72 h. The treatment of 30 mM was selected as high glucose concentration; mannitol (30 mM/l) was selected as an osmotic control. The high glucose concentration treated cells were treated with FXN (40 µM unless noted otherwise) for 24 h. DDH (10 µM) was added to the media 1 h before the cells were treated with glucose or FXN.
Cell viability studies
A cell counting kit-8 (Abcam USA) was used to measure cell viability of cardiomyocytes following the supplier’s protocol. Briefly, for cell viability assay the conversion of water soluble tetrazolium salt to water soluble formazan dye takes place due to reduction by dehydrogenases [13]. The cardiomyocytes were grown in 96-well plates for 48 h and were supplemented with various concentrations of FXN (5, 10, 20, 40, 80 µM), then the plates were incubated for 12 h in an incubator. The cells were washed and 10 µl of CCK-8 reagent was transferred to each well and incubated at 37°C for 2 h. A microplate reader (Lambda, Bio-20) was used to record the optical activity at 450 nm. Percent cell viability was calculated relative to the control (untreated cells).
Biochemical evaluation
Activity of LDH was evaluated according to its property to catalyze the reaction between lactic and pyruvic acid. The levels of markers of oxidative stress were analyzed by measuring malondialdehyde (MDA) by the TBARS method. The activity of the antioxidant enzyme SOD was done by measuring its capacity to inhibit the oxidation of oxymine. The CAT activity was assessed by measuring the decomposition of hydrogen peroxide per minute. The activity of GSHpx was assessed by measuring the decomposition rate of hydrogen peroxide and oxidation of glutathione. The assay for measuring markers of oxidative stress was done by a colorimetric method using a microplate reader. The protein was estimated by a protein estimation kit (Sigma-Aldrich USA).
Measurement of intracellular ROS
The measurement of intracellular superoxide anions was based on high performance liquid chromatographic assay and dihydroethidium fluorescence probe. The target cells (cardiomyocytes) were rinsed at least 3 times using cold Dulbecco’s phosphate-buffered saline and were then incubated with dihydroethidium (10 µM) for 20 min at room temperature. The cells were washed after incubation, to remove free dihydroethidium, the cells were viewed using a fluorescence microscope (Olympus, Japan) and images were recorded. HPLC (Waters Alliance, USA) assay was carried out for analyzing the generation of intracellular generated ROS using a dihydroethidium probe at excitation wavelength of 490 nm and emission wavelength of 596 nm [14]. The procedure was followed by incubation of cardiomyocytes in DMEM medium supplemented with dihydroethidium (10 µM) for 30 min; the cells were then harvested and were permeabilized with 0.1% Triton X100 in Dulbecco’s phosphate-buffered saline, and protein estimation was done using protein estimation kit Sigma-Aldrich (USA). About 100 µl of cell lysate was transferred to a mixture containing 0.2 mol/l of HClO4 and methanol (50 : 50 %v/v); the resultant was incubated in an ice bath for 3 h for protein precipitation. The mixture was subjected to cooling centrifugation at 4°C and 12000g for 30 min; about 100 µl of supernatant was mixed with 100 µl of phosphate buffer saline (pH 2.6). The resultant mixture was centrifuged again before subjecting it to HPLC analysis.
TUNEL assay for detection of apoptotic cells
TUNEL assay, i.e. terminal deoxynucleotidyl transferase-mediated dUTP nick end-labeling, was carried out for detection of apoptosis in cardiomyocytes. An in Situ Cell Death Detection Kit, Fluorescein (Sigma-Aldrich USA) was used for the study following the supplied instructions. Briefly, the cells were rinsed three times using Dulbecco’s phosphate-buffered saline after the defined treatments and then fixed with paraformaldehyde (4%) at 37ºC in the dark. The cells were incubated with protein kinase for 20 min at 37ºC, after which the cells were treated with H2O2 (3%) for 20 min at 37ºC. The cells were rinsed three times with phosphate buffer saline and were treated with the TUNEL detection kit. The cells were stained using 3,3′-diaminobenzidine followed by staining with hematoxylin. The images were taken using fluorescence microscopy (Olympus, Japan).
Annexin V-FITC and propidium iodide (PI) staining for detection of apoptosis
The apoptosis of cardiomyocytes was also detected by a dual staining approach using an Annexin V-FITC and PI kit with the help of a flow cytometer (Life Science Research, Bio-Rad USA). About 1 × 106 cells/well were transferred to a 96-well plate and exposed to FXN (40 µM). The cells were centrifuged and were resuspended in binding buffer to produce a volume of 100 µl, to which 5 µl of staining solution (Annexin V-FITC and PI) was added, the cells being given a gentle swirl to mix. The cells were then incubated for 20 min in the dark at 37°C. The cells were collected and to each well binding buffer (400 µl) was added followed by flow-cytometer study immediately.
Evaluation of Rac1 activity
The Rac1 activity was determined by precipitation of Rac1-GTP from cell lysates; the activity was measured by a Rac1 specific monoclonal antibody. A Rac1 activation kit (Abcam, USA) was used for the study. The cardiomyocytes were rinsed three times with Dulbecco’s phosphate-buffer saline. The proteins from the cells were extracted using a cocktail of protease inhibitor. The homogenates were centrifuged at 10000 rpm for 20 min; the supernatant was used for measuring the activity for Rac1 pulldown assay followed by total Rac1 count. About 15 µl of PAK1-PBD agarose beads were transferred to each well followed by incubation for 12 h at 4°C. The GTP-bound Rac1 was obtained by centrifugation. Western blot analysis was done for studying the expression of total and active Rac1.
Evaluation of NADP+/NADPH ratio
The ratio of NADP+/NADPH was evaluated with the NADP/NADPH Kit (ab65349) in accordance with the supplied instructions. The evaluation was based on a cycling reaction of glucose dehydrogenase in which the developed NADPH causes reduction of the probe into an intense fluorescent product. The intensity of the formed product was measured at excitation wavelength of 530 nm and emission wavelength of 585 nm, which was directly correlated to NADP+/NADPH present in the target sample.
Immunohistochemistry and evaluation of Bcl-2 and Bax
For immunohistochemical analysis, the cardiomyocytes were washed three times with Dulbecco’s phosphate-buffered saline. All the three treatment groups of cells were fixed using paraformaldehyde (4%) at 37ºC for 20 min followed by permeabilization using TritonX-100 (0.1%) for 10 min. The resultant cardiomyocytes were inactivated by endogenous peroxidase along with H2O2 (3%) for 15 min in the dark; the cells were blocked using BSA (5%) for 20 min at 37ºC. The obtained cardiomyocytes were washed three times, after which the cells were incubated at 4ºC for 12 h with anti Bcl-2 antibody at dilution 1 : 300 (Abcam, USA) and anti-Bax at dilution 1 : 200 (Abcam USA). The cells were supplemented with horseradish peroxidase conjugated IIry goat anti-rabbit IgG antibody and were incubated for 25 min. The cells were visualized using DAB and counterstained with hematoxylin dye; the slides were dehydrated with alcohol followed by a cover slip. The images were processed using Image-Pro software.
Western blot study
The cells were transferred to a 96-well plate followed by lysis using RIPA lysis buffer in an ice bath for 20 min. The obtained lysates were subjected to centrifugation at 10000 rpm for 20 min at 4°C. The proteins in the cell lysates were estimated using a protein estimation kit (Sigma-Aldrich USA). The lysates were treated with antibodies such as Bax, GSK-3β, Bcl-2, p-AMPKα, p67phox, AMPKα, p22phox, p67phox, gp91phox p47phox, pAkt, Akt, pGSK, GSK-3β (Cell Signaling, USA) and actin (Abcam, USA). The membranes were incubated along with horseradish peroxidase-conjugated IIry antibody for 60 min at 37°C. The obtained blots were developed using an ECL kit.
Ethical approval
The animals were provided by the central animal house of Wuhan Children’s Hospital. All the animal studies strictly adhered to the draft Animal protection law of the People’s Republic of China (2009) for experimental animals. The animal experiments received approval from the animal ethical committee of Wuhan Children’s Hospital, China.
Statistical analysis
The statistical analysis was done using GraphPad Prism software. All the data presented in the manuscript are shown as mean ± %RSD. The statistics was done using one-way analysis of variance (ANOVA) and Student-Newman-Keuls analysis. The values of p < 0.05 were regarded as significant.
Results
Fucoxanthin enhanced the cell viability of neonatal cardiomyocytes treated with high glucose
To evaluate the function of FXN in cell survival, we studied cell viability of Iry cardiomyocytes obtained from neonatal rats treated with various concentrations of glucose ranging from 5 to 50 mM/l of D-glucose for 72 h (Figure 1 A). The results suggested that the cell viability decreased with increasing concentration of glucose. At 30 mM there was a significant fall; hence we chose this concentration for our experiments ahead and described it as high glucose media. We incubated the cardiomyocytes in high glucose media for 24 h and it was observed that there was a significant fall in viability (p < 0.05) (Figure 1 B). In the experiment mannitol (30 mM/l) was selected as a control but failed to mimic the effect of d-glucose. The cardiomyocytes were exposed to FXN in the concentration ranging from 5, 10, 20, 40 and 80 µM in high glucose media (30 mM/l) for 24 h; the results suggested that the dose of FXN at 40 and 80 µM caused a significant increase in cell viability and decreased the release of lactate dehydrogenase in the media (p < 0.05) (Figures 1 C, D). Lactate dehydrogenase is an enzyme present in the cytosol of the animal cells; it is an oxidoreductase enzyme. The enzyme is stable and is an indicator to study the damage and toxicity of the cells and tissues. The release of LDH indicates loss of membrane integrity, suggesting cell death. The results of LDH suggested that FXN could protect the cardiac cells from injury mediated by high glucose. In the study, the cardiomyocytes were cultured in a high glucose environment and were treated with 40 µM of FXN.
Figure 1
Fucoxanthin increases the cell viability in Iry neonatal rat cardiomyocytes treated with high glucose. A – The cell viability decreased significantly when the cells were exposed to high glucose environment (30 mM/l). B – The cell viability decreased significantly when the incubation time increased to 24 h in 30 mM/l glucose, 30 mM/l mannitol was adopted as an osmotic control. C – Addition of fucoxanthin (40 and 80 µM/l) increased the cell viability in high glucose treated cardiomyocytes. D – Fucoxanthin (40 and 80 µM/l) decreased the release of LDH in the incubating medium of cardiomyocytes exposed to high glucose. *P < 0.05 compared to control, #p < 0.05 compared to high glucose treated rats (n = 10)
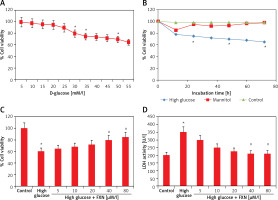
Fucoxanthin decreased oxidative stress in Iry neonatal cardiomyocytes treated with high glucose media.
The cellular levels of reactive oxygen species in cardiomyocytes were determined by dihydroethidium. The results (Figures 2 A, B) suggested that the red fluorescence intensity increased in cardiomyocytes cultured under high glucose media versus control (p < 0.05). The exposure to FXN 40 µM showed significant inhibition in generation of ROS (p < 0.05) versus the cells cultured only in high glucose media without FXN. The fluorescence by dihydroethidium was further evaluated by chromatographic assay; the results (Figure 2 C) suggested that the levels of ROS were elevated significantly in cells treated with high glucose, whereas a reverse pattern was seen in cell treated with FXN (p < 0.05).
Figure 2
Fucoxanthin halted the generation of reactive oxygen species in Iry cardiomyocytes treated with high glucose. DHE and fluorescent probe were used for measuring intracellular levels of reactive oxygen species in cardiomyocytes. A – Cardiomyocytes staining with DHE showing representative fluorescence images (400×, scale bar: 20 µm). B – Intensity of red fluorescence (quantitative analysis). C – HPLC analysis for dihydroethidium fluorescence, fucoxanthin 40 µM. *P < 0.05 compared to control, #p < 0.05 compared to high glucose treated group (n = 10)
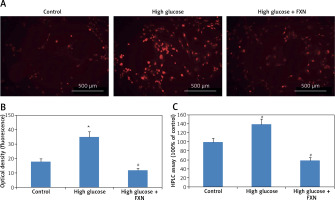
We then evaluated the role of NADPH oxidase in the increase in production of ROS in cells cultured in high glucose media. The literature suggests that the enzyme NADPH oxidase is a complex structure made up of subunits such as p22phox, p67phox, p47phox, gp91phox and Rac1. We performed Rac1 activity assay; the results (Figures 3 A, B) demonstrated that the Rac1 activity and levels of gp91, p67phox and p47phox were markedly upregulated due to the high glucose environment compared to control cells (p < 0.05). In contrast, the FXN treatment caused a significant reversal in Rac1 activity along with expression of gp91, p67phox and p47phox compared to cells cultured only in high glucose media without FXN (p < 0.05). These results of our experiment confirmed that FXN decreased the levels of NADPH oxidase, which further suppresses the generation of ROS in the neonatal cardiac cells receiving treatment with high glucose.
Figure 3
Fucoxanthin blocked NADPH oxidase subunits in Iry neonatal rat cardiomyocytes treated with high glucose. A – Fucoxanthin decreased the activity of Rac1 in high glucose treated cardiomyocytes. B – Fucoxanthin suppressed the levels of NADPH oxidase subunits gp91phox, p47phox, p67phox, whereas the levels of p22phox remained unaltered in cardiomyocytes treated with high glucose. Fucoxanthin 40 µM. C – Quantitative results showing expression of GTP-Rac1 against total Rac1. D – Quantitative results showing expression of NADPH oxidase subunits gp91phox, p47phox and p67phox and p22phox. *P < 0.05 compared to control, #p < 0.05 compared to high glucose media treated group (n = 6)
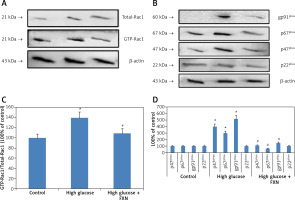
The burden of oxidative stress was further evaluated by studying the activity of endogenous antioxidant enzymes. The biochemical evaluation in cardiomyocytes (Figures 4 A–E) demonstrated that high glucose media resulted in increased formation of MDA and decreased the activity of anti-oxidant enzymes SOD, CAT, GSHpx and GSH compared to control cells (p < 0.05). Treatment of FXN 40 µM resulted in inhibition of lipid peroxidation and improved the antioxidant status by increasing the activity of antioxidant enzymes SOD, CAT and GSHpx; the treatment also elevated the levels of GSH in cells compared to cells cultured only in high glucose media without FXN (p < 0.05).
Figure 4
Fucoxanthin suppressed the levels of MDA, elevated the activity of SOD, CAT, and GSH-px, and maintained the content of GSH in Iry neonatal rat cardiomyocytes treated with high glucose media. A – Biochemical estimation of markers of oxidative stress, MDA, B – SOD, C – catalase, D – GSH-px respectively. E – The content of GSH was measured by biochemical analysis. Fucoxanthin: 40 µM. *P < 0.05 compared to control, #p < 0.05 compared to high glucose media treated group (n = 10)
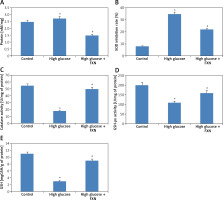
Fucoxanthin protects neonatal cardiomyocytes against high glucose-induced apoptosis
The apoptosis study in cells was done by TUNEL staining as well as by flow cytometry. The results of TUNEL staining (Figures 5 A, B) demonstrated an increased number of apoptosis positive cells with brown staining in high glucose media treated cells compared to the control. The treatment with FXN 40 µM protected the cardiac cells from glucose-induced apoptosis.
Figure 5
Fucoxanthin blocked high glucose-mediated apoptosis in Iry neonatal rat cardiomyocytes treated with high glucose. A – TUNEL staining of apoptosis induced cardiomyocytes (400×, scale bar: 20 µm). B – Apoptosis rate % TUNEL staining of cardiomyocytes. C – PI and Annexin V staining for apoptotic cells by flow cytometer. Fucoxanthin 40 µM. *P < 0.05 compared to control, #p < 0.05 compared to high glucose media treated group (n = 6)
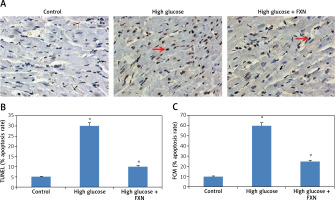
An apoptosis study by Annexin-V and propidium iodide (PI) by flow cytometry was also done (Figure 5 C). The pattern Annexin V (+)/PI (–) suggested presence of a cell population exhibiting early apoptosis, whereas Annexin (+)/PI (+) indicated a cell population with delayed apoptosis. The results indicated that both cell populations, i.e. Annexin V (+)/PI (–) and Annexin (+)/PI (+), significantly increased in cells incubated in high glucose media without FXN compared to the control (p < 0.05). The treatment with FXN 40 µM resulted in a significant decrease in both cell populations compared to cells incubated in high glucose media without FXN (p < 0.05).
Fucoxanthin regulates the levels of Bcl-2 and Bax in neonatal cardiomyocytes treated with high glucose
After apoptosis studies, we evaluated the levels of Bax (pro-apoptotic protein marker) and Bcl-2 (anti-apoptotic marker) as important apoptotic protein markers, further confirming the role of FXN against apoptosis. We extended our study to find out whether treatment with FXN suppressed apoptosis via regulating both of these important proteins. The results of immunohistochemistry (Figures 6 A, B) suggested that high glucose media caused significant up-regulation in the levels of Bax but down-regulated the levels of Bcl-2 compared to control cardiomyocytes (p < 0.05). We further observed that FXN 40 µM caused significant suppression in expression of Bax-2 and up-regulation of Bcl-2 in cardiac cells compared to cells exposed to high glucose media without FXN (p < 0.05). The results showing expression of both the apoptotic proteins by western blot were parallel to the immunohistochemical findings (Figure 6 C).
Figure 6
Fucoxanthin modulated the expression levels of Bax and Bcl-2 in Iry neonatal rat cardiomyocytes treated with high glucose. A – Immunohistochemical staining of Bcl-2 and Bax in cardiomyocytes in control, high glucose and FXN treated cells (400×, scale bar: 20 µm). B – Immunostaining of Bax and Bcl-2 in control, high glucose and FXN treated cells. C – Western blot analysis for expression of Bcl-2 and Bax in cardiomyocytes. Fucoxanthin 40 µM. *P < 0.05 compared to control, #p < 0.05 compared to high glucose media treated group (n = 6)
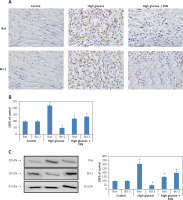
Effect of fucoxanthin on AMPK and Akt/GSK-3β pathway in neonatal cardiomyocytes treated with high glucose
The Akt/GSK-3β pathway is regarded as an important regulator for cell proliferation, survival and apoptosis. The findings of our study suggested that expression levels of both p-Akt and GSK-3β were down-regulated significantly in cardiomyocytes cultured in high glucose media compared to control cells; importantly we found that treatment with FXN 40 µM caused no changes in levels of p-Akt and GSK-3β in cardiomyocytes treated with high glucose (Figure 7 A). The outcomes confirmed no involvement of Akt/GSK-3β axis in the protective effect of FXN against high glucose induced apoptosis of cardiac cells.
Figure 7
Effect of fucoxanthin on expression levels of AMPK and Akt/GSK-3β in Iry neonatal rat cardiomyocytes treated with high glucose. A – Expression of p-Akt and GSK-3β proteins by western blot analysis. B – p-AMPK analysis by western blot study. DDH (10 µM) could abolish the elevated levels of pAMPK levels by fucoxanthin in cardiac cells treated with high glucose. Fucoxanthin 40 µM. *P < 0.05 compared to control, #p < 0.05 compared to high glucose media treated group, &p < 0.05 compared to high glucose + FXN treated group of cells (n = 6)
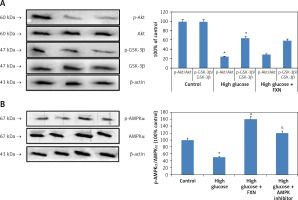
Upon evaluation of AMPK, we found that treatment with FXN significantly enhanced the p-AMPKα in cardiomyocytes compared to those cultured in high glucose media (Figure 7 B). Treatment with DDH (10 µM) (AMPK inhibitor) was found to reverse the effects of FXN-induced elevation of pAMPKα; it decreased the levels of pAMPKα significantly compared to cells cultured in high glucose media with FXN (p < 0.05). In parallel to these findings, FXN failed to modulate the activity of Rac1, NADPH and the expression of other subunits (gp91phox, p47phox and p67phox) in the presence of DDH (10 µM) (Figures 8 A–C) (p < 0.05). In addition, we observed that the levels of Bcl-2 and Bax which were regulated by FXN earlier were also reversed by the DDH (Figure 9 A) (p < 0.05). The findings hence confirmed that, when combined, FXN with DDH results in blockade in anti-apoptotic activity of FXN in glucose-induced apoptotic neonatal cardiomyocytes (Figure 9 B) (p < 0.05).
Figure 8
Blockade of AMPK abolished the effect of fucoxanthin on the activity of NADPH oxidase and expression its subunit in Iry neonatal rat cardiomyocytes treated with high glucose. A – Fucoxanthin suppressed the activity of Rac1, which was blocked by DDH. B – Fucoxanthin along with DDH reversed the expression levels of NADPH oxidase subunits p67phox, p47phox, and gp91phox. C – DDH reversed the inhibition of NADPH activity mediated by fucoxanthin. Fucoxanthin 40 µM. *P < 0.05 compared to control, #p < 0.05 compared to high glucose media treated group, &p< 0.05 compared to high glucose + FXN treated group of cells (n = 6)
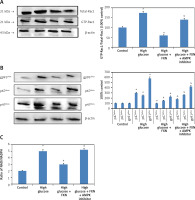
Figure 9
Inhibition of AMPK aggravated apoptosis in Iry neonatal rat cardiomyocytes treated with high glucose. A – DDH blocked the effect of fucoxanthin on expression of Bcl-2 and Bax. B – Image showing apoptosis in myocytes by TUNEL staining. Quantitative analysis of cardiomyocytes subjected to TUNEL staining. Fucoxanthin 40 µM. *P < 0.05 compared to control, #p < 0.05 compared to high glucose media treated group, &p < 0.05 compared to high glucose + FXN treated group of cells (n = 6)
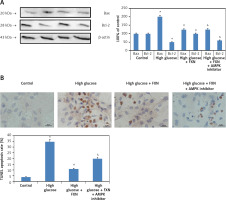
Discussion
As a distinctive feature of diabetes, the hyperglycemic condition is one of the important factors responsible for structural, metabolic and functional alterations in the cardiac tissues, resulting in a condition called diabetic cardiomyopathy (DC). Abnormal glucose metabolism, which is the main feature of diabetes, can activate a series of abnormal processes in development and progression of DC [15–17]. In the present work, the neonatal cardiomyocytes when cultured in high glucose media (30 mM/l) for 24 h resulted in increased apoptosis and cell injury. The results were in parallel to previous findings which suggested that apoptosis of cardiomyocytes is associated with blood glucose levels [18]. Apoptosis is programmed cell death which is vital for maintaining homeostasis. Increase in apoptosis of cardiomyocytes leads to failure of contractile cardiac tissues, which further causes cardiac remodeling, decreased contractility, hypertrophy and failure of the heart [19, 20]. Studies have recently confirmed the importance of cardiomyocyte apoptosis in development of DC [21, 22]. A study recently correlated increased apoptosis to poor cardiovascular outcomes [23]. In the present study, treatment of FXN protected the cardiomyocytes against glucose-mediated apoptosis and cell injury. Since the process of programmed cell death, i.e. apoptosis, is modulated by number of regulatory proteins, further in our study we analyzed the expression of apoptosis regulatory proteins such as Bax and Bcl-2. As predicted, the treatment with FXN reversed the Bax/Bcl-2 ratio in neonatal cardiac cells exposed to high glucose conditions.
Elevated oxidative stress is a major factor in development of DC. NADPH oxidase is a membrane bound enzyme and is one of the main sources of ROS in the cardiac cells. The enzyme NADPH oxidase is made up of multiple proteins or the subunits which are necessary for activating the enzymes in the cells. The expression of these NADPH oxidase subunits is altered under various pathological conditions [24]. In the present work, the exposure of cardiomyocytes to a high glucose environment resulted in up-regulation of gp91phox, p67phox, p47phox and Rac1 and also elevated the activity of NADPH oxidase. We also found that a high glucose environment impaired the status of the endogenous anti-oxidant system. High glucose suppressed the activity of antioxidant enzymes such as CAT, SOD, GSHpx and contents of GSH. The elevated glucose conditions enhanced lipid peroxidation. Fucoxanthin is a carotenoid having special functional groups which include 5,6-monoepoxide structure and an allenic bond. FXN has been reported to show antioxidant activity by curbing oxidative stress [9]. In the present work, FXN suppressed the production of ROS generated from NADPH oxidase in the neonatal cardiomyocytes cultured in an elevated glucose environment. The findings are in agreement with previous studies which suggest that FXN blocks NADPH-mediated reactive oxygen species in 3T3-L1 adipocytes [25] and neuronal cells [26]. FXN has been found to elevate the activity of antioxidant enzymes such as SOD, CAT and GSHpx, and also it improves content of GSH at the cellular level [27]. In our present work, FXN attenuated oxidative stress mediated by a high glucose environment by modulating ROS, as evidenced by elevation of activity of SOD, CAT and GSHpx. The treatment improved levels of GSH and suppressed MDA.
In the present work we studied generation of ROS mediated by NADPH oxidase only, and the role of FXN in other sources producing reactive oxygen species such as mitochondria in cardiomyocytes still remains to be unexplored. Here we identified the pathway responsible for the beneficial effect of FXN in cardiac cells exposed to high glucose. AMPK is vital to maintain cellular stores of energy by shifting it to pathways which produce ATP. Activation of AMPK has been found to protect against a number of cardiac disorders associated with apoptosis via insulin dependent and independent pathways [28, 29]. In our study, we demonstrated that activation of AMPK is involved in the protective action of FXN on neonatal cardiomyocytes via suppressing the oxidative stress mediated by a high glucose environment. Our findings were in agreement with an earlier study which suggested that FXN exerts a protective effect against high glucose induced oxidative stress in human umbilical cells and in a zebrafish model [30]. In a study, it was confirmed that activation of NADPH oxidase due to the hyperglycemic condition in cardiac cells is independent of glucose metabolism [31, 32]. Our findings provided novel evidence that FXN exerts a protective effect on cardiomyocytes in DC.
In conclusion, our study established that FXN when exposed to Iry neonatal cardiomyocytes subjected to a high glucose environment exerted a protective effect by halting apoptosis via blocking NADPH-induced ROS production and improving the activities of antioxidant enzymes of cardiomyocytes. We also confirmed that these effects of FXN involve the AMPK cascade. Altogether our findings confirm the therapeutic potential of FXN in treating diabetes-associated cardiac diseases.