Introduction
One of the many anthraquinones with potential antimutagenic activity is anthraflavic acid (2,6-dihydroxyanthraquinone). This compound has been shown to markedly inhibit the mutagenicity of 7,8-dihydroxy-9,10-epoxybenzo[a]pyrene, the ultimate mutagen/carcinogen of benzo[a]pyrene, in the Ames test, presumably by the formation of non-genotoxic products [1]. Amylases are predominant hydrolase enzymes that hydrolyze glycosidic bonds present in starch molecules and produce dextrins and oligosaccharides. Amylases are members of glycoside hydrolase family 13 (GH-13). In starch, α-1,4-glycosidic linkages are hydrolyzed by amylases, so they are known as glycoside hydrolases [2, 3].
Theoretical investigations are multifaceted procedures that can be used as parallel examinations for experimental research. The value of the experimental results can be boosted by adopting such strategies to gain greater insight into the results [4]. One of these analytical methods is docking calculation. It can determine some parameters which are required for identifying the mechanisms and interactions for a ligand-biomolecule complex. These computations could support the outcomes of empirical investigations. The prediction of inhibition mechanisms is one of the helpful duties for molecular docking [5]. The obtained results of the molecular docking study could be further evaluated using the molecular mechanics/generalized Born surface area (MM/GBSA) approaches [6].
In the present study, also the properties of anthraflavic acid against common breast carcinoma cell lines, i.e. MCF-7, CAMA-1, SK-BR-3, MDA-MB-231, AU565 [AU-565], Hs 281.T, and anti-diabetic action were evaluated with molecular docking.
Material and methods
Material
Phosphate buffer solution (PBS), 4-(dimethylamino)benzaldehyde, Ehrlich solution, dimethyl sulfoxide (DMSO), Dulbecco’s Modified Eagle Medium (DMED), hydrolysate, decamplmaneh fetal bovine serum, borax-sulfuric acid mixture, 2,2-diphenyl-1-picrylhydrazyl (DPPH), and antimycotic antibiotic solution all were obtained from Sigma-Aldrich company of USA.
Anti-human breast carcinoma properties of anthraflavic acid
In this research, we used human umbilical vein endothelial cells (HUVECs) to determine the cytotoxicity potential of anthraflavic acid (CAS number: 84-60-6, molecular weight: 240.21, EC number: 201-544-3, MDL number: MFCD00001228, and PubChem substance ID: 24891386 taken from Sigma) using 3-(4,5-dimethylthiazol-2-yl)-2,5-diphenyl-2H-tetrazolium bromide (MTT) assay. Also, the in vitro anti-breast carcinoma effects of biologically synthesized anthraflavic acid against MCF-7, CAMA-1, SK-BR-3, MDA-MB-231, AU565 [AU-565], and Hs 281.T cancer cell lines were evaluated.
These cell lines were plated on DMEM media using 96-well plates with antimycotic solution, 10% FBS, streptomycin and penicillin. Cells number in each plate-based well was 10,000, incubated at 37°C for 24 h and treated with anthraflavic acid with different dilutions (0–1000 μg/ml) and incubated for 24 h. Then, 5 mg/ml of MTT was added to all wells, and finally incubated at 37°C for 4 h. After the absorbance evaluation at 540 nm, the cell viability percentage was calculated from the following equation [7]:
After collecting data, Minitab statistical software was used for statistical analysis. Evaluation of antioxidant results in a completely randomized design and comparison of means was performed with the Duncan post-hoc test with a maximum error of 5%. To measure the percentage of cell survival in factorial experiments with the original design of completely randomized blocks and compare the means, the Duncan post-hoc test with a maximum error of 5% was used. The 50% cytotoxicity (IC50) and 50% free radical scavenging (IC50)) were estimated with ED50 plus software (INER, V: 1.0). Measurements were reported as mean ± standard deviation.
Enzyme method
Inhibition of α-amylase activity was carried out as described by Adefegha and Oboh (2012) [8]. Briefly, 200 μl of appropriate dilutions of the Justicia carnea aqueous leaf extract (JCAE) (100–800 μg/ml) and 500 μl of α-amylase (EC 3.2.1.1, 0.5 mg/ml, prepared with 0.02 M sodium phosphate buffer (pH 6.9) with 0.006 M sodium chloride) were incubated at 25°C for 10 min. Then, 500 μl of 1% starch solution in 0.02 M sodium phosphate buffer (pH 6.9 with 0.006 M sodium chloride) was added to each tube [9]. The reaction mixture was incubated at 25°C for 10 min and stopped with 1.0 ml of dinitrosalicylic acid color reagent. Thereafter, the mixture was incubated in a boiling water bath for 5 min and cooled to room temperature. The reaction mixture was then diluted to 10 ml with distilled water, and absorbance was measured at 540 nm against distilled water blank. The experiment was repeated using acarbose as a standard antidiabetic drug (positive control). The α-amylase inhibitory activity was expressed as percentage inhibition [10].
Molecular docking study
In addition to some empirical investigations, the biological characteristics of chemical compounds could be examined using analytical methods such as a molecular docking study. The enzymes used in this study were human pancreatic α-amylase (PDB ID: 1HNY) [11]. The biological activities of anthraflavic acid and acarbose against this enzyme were evaluated using molecular docking. After obtaining the enzyme structure from the PDB database (http://www.rcsb.org/pdb), it was prepared with the protein preparation module of the Schrödinger Suite [12]. The hydrogen atoms were added to the enzyme structure, and the missing side chains were replaced using primers. The water molecules beyond 5Å from het groups were removed, and an H-bond network was created using the optimization step of the module. The minimization step was then applied for the structure using optimized potentials for liquid simulations (OPLS3e) force field. The binding sites of the enzyme were predicted using the SiteMap of Schrödinger to find the active sites of the structure [13]. The SDF files of anthraflavic acid and acarbose were obtained from the PubChem database and the correct molecular geometrics were generated utilizing the LigPrep module of Schrödinger [14]. The Epic module was used to produce protonation states. Lastly, molecular docking was performed employing Glide of the Schrödinger Suite.
Binding free energy calculations
The binding free energy of the ligand-enzyme complex was calculated conducting the MM/GBSA method (Schrödinger Release 2020-2: Prime, Schrödinger, LLC, New York, NY, 2020-2) [15]. The solvation model of VSGB and OPLS3e force field were used for the calculations [16]. The equation of binding free energy is displayed here: ΔG bind = G complex – (G protein + G ligand).
Where ΔG bind indicates the binding free energy, G complex is the binding free energy. The G protein and G ligand indicate the binding free energy of the protein and ligand, respectively.
Results and discussion
Cancer results
Cancer is now one of the leading causes of death worldwide. Existing treatments have not been able to meet the treatment needs for various types of cancer. Therefore, the use of new technologies in the prevention and treatment of cancer can be helpful. Extensive research on molecules has been conducted in recent years [17, 18]. The advent of biotechnology has had a profound effect on many areas of healthcare and scientific research. Common cancer treatments, including chemotherapy, radiation and surgery, may reduce the size of the tumor, but the effect of these methods is transient and has no positive effect on patient survival. Therefore, replacing more effective, more specific therapies with fewer side effects with higher anti-cancer activity is a dominant issue in clinical oncology [19]. The gradual maturation of biotechnology has been considered not only for treating cancer but also for a wide variety of applications, especially for drug delivery and diagnostic and imaging cases. There are many types of molecules available and choosing the right carriers according to demand is a key issue [20–22]. Molecules are very close in size to biological molecules and can easily penetrate the cell; for this reason, one of the goals of biotechnology is to mount molecules and drugs on molecules and transfer them to the target cell [23, 24]. It is also possible to create different surface properties for molecules by attaching protective ligands to increase the molecules’ resistance to the immune system and increase their presence in the bloodstream, and even binding ligands to specifically bind the molecules to the target tissue [25–29].
One of the cytotoxicity test methods to measure the rate of cell death is the MTT method, which is based on the formation of formazan dye by reducing the substance MTT (dimethyl thiazole 2 and 5 diphenyltetrazolium bromide) or other tetrazolium salts [7]. Through breaking of the MTT tetrazolium ring by mitochondrial enzymes in living cells, insoluble purple formazan crystals are formed. The formation of these crystals indicates the activity of respiratory chain enzymes and is a measure of cell viability. By measuring the amount of absorption by spectrophotometry at specific wavelengths, the number of living cells can be determined. This test is performed according to ISO 10993-5 and its purpose is in vitro evaluation of cytotoxicity. The cytotoxicity test is performed according to the ISO10993-5 standard and in three ways: NRU test, CFU test, MTT test and XTT test. The most common method for assessing cytotoxicity is to measure cell survival by MTT [7]. The MTT method is based on the intensity of dye produced by the mitochondrial activity of cells, measured at a wavelength of 540 to 630 nm and directly proportional to the number of living cells; the increase or decrease in the number of living cells is linearly related to the activity of cell mitochondria. MTT tetrazolium dye is revived in active (metabolically) cells. Mitochondrial dehydrogenases in living cells produce NADH and NADPH, leading to an insoluble purple precipitate called formazan. This precipitate can be dissolved by isopropanol or dimethyl sulfoxide [7]. Dead cells, on the other hand, are unable to perform this conversion due to the inactivity of their mitochondria and therefore do not show a signal. In this method, dye formation is used as a marker for the presence of living cells. In recent years, MTT testing has been the most important measurement method to evaluate the toxicity and anti-cancer effects of molecules [7].
In this study, cells treated with different concentrations of anthraflavic acid were assessed by MTT assay for 48 h as regards the cytotoxic properties on normal (HUVEC) and breast malignancy cell lines i.e. MCF-7, CAMA-1, SK-BR-3, MDA-MB-231, AU565 [AU-565], and Hs 281.T (Figures 1, 2).
Figure 1
Anti-human breast adenocarcinoma properties (cell viability (%)) of anthraflavic acid (concentrations of 0-1000 μg/ml) against human breast adenocarcinoma (MCF-7 (A), CAMA-1 (B), SK-BR-3 (C), and MDA-MB-231 (D)) cell lines. Numbers indicate the percentage cell viability at concentrations of 0–1000 μg/ml of anthraflavic acid for several human breast adenocarcinoma cell lines
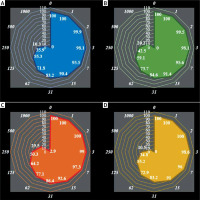
Figure 2
Anti-human breast adenocarcinoma properties (cell viability (%)) of anthraflavic acid (concentrations of 0–1000 μg/ml) against human breast adenocarcinoma (AU565 [AU-565] (A) and Hs 281.T (B)) cell lines. Numbers indicate percentage cell viability at concentrations of 0–1000 μg/ml of anthraflavic acid for several human breast adenocarcinoma cell lines
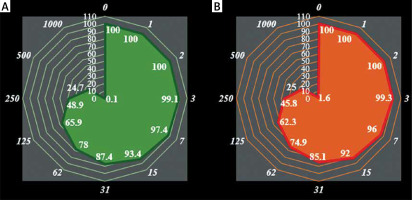
The viability of the malignant breast cell line decreased dose-dependently in the presence of anthraflavic acid. The IC50 values of anthraflavic acid were 159, 193, 253, 156, 241, and 218 μg/ml for MCF-7, CAMA-1, SK-BR-3, MDA-MB-231, AU565 [AU-565], and Hs 281.T cell lines, respectively (Table I).
Table I
IC50 of anthraflavic acid in anti-human breast adenocarcinoma test
Variable | MCF-7 | CAMA-1 | SK-BR-3 | MDA-MB-231 | AU565 [AU-565] | Hs 281.T |
---|---|---|---|---|---|---|
IC50 [μg/ml] | 159 ±0 | 193 ±0 | 253 ±0 | 156 ±0 | 241 ±0 | 218 ±0 |
The absorbance rate was evaluated at 570 nm, representing viability on a normal cell line (HUVEC) even up to 1000 μg/ml for anthraflavic acid (Table I and Figures 1, 2).
Enzymes results
The enzymatic starch digestion process as affected by α-amylase inhibitors is complicated. To date, most of the factors affecting the inhibition have been revealed, including particle size, crystallinity, composition and structure of starch. Hydrogen bonds and a conjugated π-system were critical for flavonoid inhibitors to control starch digestion [30]. However, the contribution of hydrophobic interaction to the inhibitory mechanism has been scarcely studied. The important purpose of this study is to determine an efficacious, selective and more potent inhibitor for the enzyme α-amylase [31]. Hence, inhibition analysis of this enzyme was recorded with anthraflavic acid to control diabetic complications. The IC50 result for this compound was 198.30 nanomolar. Type 2 diabetes mellitus (T2DM) is characterized by elevated blood glucose levels and can lead to serious complications such as nephropathy, neuropathy, retinopathy and cardiovascular disease [32].
Docking results
The biological activities of anthraflavic acid as an inhibitor for α-amylase were investigated with molecular docking calculations. Acarbose was also considered as a validated standard inhibitor for α-amylase. The docking pose of anthraflavic acid is presented in Figure 3, and Figure 4 shows the interactions between various residues of the enzyme and anthraflavic acid. Figure 4 shows that an oxygen atom of the ligand has created two hydrogen bonds with Trp59 and Gln63. This oxygen atom is a hydrogen bond donor, forming hydrogen bonds with the oxygen of Trp and nitrogen of Gln. Trp59 is one of the common residues for anthraflavic acid and acarbose that has created interaction with the ligands. Another residue that has hydrophobic contact with anthraflavic acid is Glu233. This interaction is an important contact since Glu233 is a crucial member of the enzyme active site [33]. The presence of this contact among hydrophobic contacts could lead to inhibitory activity of this compound. Figure 5 shows the docking pose of acarbose among α-amylase residues, and their interactions are presented in Figure 6. As can be seen, acarbose is very active when contacting the enzyme and has created several hydrogen bonds and hydrophobic contacts. The parameters obtained from docking calculations are shown in Table II. The docking score indicates the binding affinity, which is the most important parameter [34]. The molecule’s efficiency is another essential parameter, numerically calculated and shown with the glide ligand efficiency. Glide evdw and glide ecoul are Van der Waals and Coulomb energy, respectively. These two parameters are interaction-related, and glide energy is calculated as a modification of their energy. The next parameter is glide emodel, which shows the value of the interaction pose [35]. Some of the obtained results of free binding energy are presented in Table III. As can be clearly seen, the docking scores have a positive correlation with the reported IC50 of the compounds. This correlation is further supported by the value of binding energy, which is –19.36 for anthraflavic acid and –11.82 for acarbose. It can be concluded from these results that the predicted docking pose is reliable [36]. Due to the values presented in Table III, coulomb energy has a crucial role in the binding energies between the compounds and α-amylase. However, binding energies such as H-bond, lipophilic energy, covalent energy, and Van der Waals energy are important parameters. It is evident that all of these parameters have a key role in the interactions between the molecule and enzymes. Based on the results obtained from these molecular docking investigations, like acarbose, anthraflavic acid could be considered as a potential inhibitor for α-amylase.
Table II
Parameters obtained from molecular docking calculations
Table III
The results obtained from the binding free energy calculations
Parameter | ΔGbind | ΔGbind Coulomb | ΔGbind H-bond | ΔGbind Lipo | ΔGbind vdW | ΔGbind covalent |
---|---|---|---|---|---|---|
Anthraflavic acid | –19.36 | –16167 | –320 | –1315 | –2819 | 1833 |
Acarbose | –11.82 | –16047 | –324 | –1334 | –2858 | 1862 |
Figure 4
Interactions of anthraflavic acid and α-amylase. Green dashed lines indicate hydrogen bonds, and semicircles show hydrophobic contacts
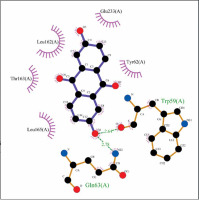
Figure 6
Interactions of acarbose and α-amylase. Green dashed lines indicate hydrogen bonds, and semicircles show hydrophobic contacts
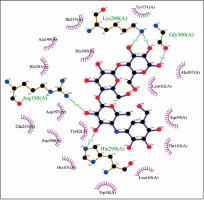
In conclusion, in this research, the viability of a malignant breast cell line was reduced dose-dependently in the presence of anthraflavic acid. The IC50 values of anthraflavic acid were 159, 193, 253, 156, 241, and 218 μg/ml for MCF-7, CAMA-1, SK-BR-3, MDA-MB-231, AU565 [AU-565], and Hs 281.T cell lines, respectively. After the clinical study, anthraflavic acid can be utilized as an efficient drug in treating breast carcinoma in humans.