Introduction
Spinal cord injury (SCI) is reported to be one of the commonest and most damaging injuries encountered by neurosurgeons. SCI can lead to permanent disability such as loss of sensation, loss of movement and paralysis. Treating SCI remains one of the biggest challenges faced by neurologists. Although many treatment approaches have been explored, they have shown limited success so far [1].
The molecular mechanisms involved in SCI are complicated and involve multiple signaling pathways. The post-traumatic inflammatory response is one of the mechanisms that contribute to progressive tissue damage after SCI. After SCI, inflammatory mediators such as cytokines and eicosanoids accumulate at the injury site, and cells such as macrophages, neutrophils and lymphocytes infiltrate rapidly at the injury site, leading to progression of SCI. Tumor necrosis factor α (TNF-α) is a important mediator of inflammation contributing to cell death in neurodegenerative disorders. Increased levels of TNF-α in the injured spinal cord have been a feature hours after injury [2, 3].
MicroRNAs (miRs) are noncoding, endogenous and single stranded RNAs made up of 22 nucleotides. MicroRNAs are responsible for regulating the expression of a number of genes through RNA-mediated silencing complexes. miRs direct the genes to mRNAs, where they can inhibit the process of translation, causing destructive cleavage [4]. About 6% of the genes are reported to be regulated by miRs [5]. As a result, miRs may significantly affect the signaling inflammatory pathways, leading to pathologies after spinal cord injury. Hence, miRs could become potential and novel targets for treating spinal cord injury.
Evidence suggests that a number of miRs are expressed in the central nervous system (CNS) [6, 7]. Recently a few miRs have been identified to be involved in a number of neurological problems including trauma-mediated CNS injury and neurodegenerative diseases [8–14]. In previous research it was demonstrated that miR-19a is over-expressed and was involved in the regulation of neuropathic pain in rats [8]. In another report, miR-19a was found to be upregulated in ischemic stroke and was also declared to be a novel biomarker [9]. In a study presented by Ge et al., miR-19a attenuated cerebral ischemic injury by targeting Adiponectin receptor protein 2 (ADIPOR2), suggesting it as a novel target for treating ischemic brain injury [10]. In addition, some studies have confirmed the involvement of miRs in the pathogenesis of spinal cord injury in animal models [11–13]. Recently TNF-α, a member of the Fas ligand gene (FasL), has been identified to be a favorable target of miR-19a [14]. In addition, miR-19a has also been found to exert a protective effect on cardiac tissues against hypoxia-mediated injury [15]. However, the role of miR-19a in spinal cord injury remains unexplored. Here we postulate that as miR-19a is a novel biomarker in ischemic stroke and neuropathic pain, it could be an interesting target in spinal cord injury.
In the present work, we performed microarray analysis for the expression of various microRNAs in a rat model of contusion SCI. We found that miR-19a was one of the highly deregulated miRs after SCI. Firstly, it was demonstrated that inhibition of miR-19a using an antagomir aggravated the functional deficit, decreased the protection of spinal cord tissue and elevated the number of apoptotic cells. The pro-apoptotic genes such as PDCD4, FasL and PTEN which are reported to be the direct targets of miR-19a are studied in many disorders and cell types [16–18].
PDCD4 only is defined as Programmed cell death-4; previously it has been reported to be upregulated under apoptotic inducing conditions. Studies have suggested that PDCD4 acts as a tumor suppressor and also as a translation inhibitor. PDCD4 halts the progression and invasion of tumor, and inhibits translation of specific mRNA via binding mRNA molecules and eukaryotic initiation factor 4A. In addition, the expression of PDCD4 is lost or inhibited in some tumors [19]. Fas is expressed by a number of normal cells, such as colon cells. Also these cells are found to be sensitive to Fas-induced apoptosis. Expression of Fas has been reported to be upregulated in a spinal cord injury animal model [20]. PTEN, i.e. phosphatase and tensin homolog, has also been studied extensively. PTEN can lead to dephosphorylation of both inositol lipids and proteins. A study recently suggested that the levels of PTEN were upregulated significantly after the animals were subjected to spinal cord injury [21].
In the present study knockdown of miR-19a in vivo after spinal cord injury upregulated the levels of PTEN and FasL in the cord tissue, but failed to alter the levels of PDCD4. Therefore, the findings of our study suggested that miR-19a can play a potential role in the pathogenesis of SCI.
Material and methods
Experimental animals
For the study, adult male Sprague-Dawley (SD) rats weighing 180–220 g were used. The animals were supplied by the animal house of The Second Affiliated Hospital of Nanchang University, China. All the experimental protocols were approved from the animal ethical committee of The Second Affiliated Hospital of Nanchang University China; the approval number was NU00748A19. The animals were housed in separate cages and maintained under controlled environmental conditions of temperature and humidity; the animals were subjected to a 12 h dark and light cycle and were provided with free access to food and water.
Development of contusion moderate SCI model
The animals were subjected to anesthesia with sodium pentobarbital (40 mg/kg) (9 mg/ml). The rats were subjected to laminectomy at T10 (vertebra 10). The contusion injury (moderate) was done by modified Allen’s weight drop instrument; the setup consisted of an eight gram weight kept at a height of 40 mm. The sham operated rats underwent laminectomy and no other procedure.
Experimental design
The study was divided into two parts; in the 1st part of the study miR microarray analysis was done. Microarray analysis was done to examine the patterns of miR expression on the 1st and the 3rd day after SCI. The animals were divided into 4 groups, named the sham-operated group (day 1, i.e. group 1, and day 3, i.e. group 2, n = 3/group), SCI induced (day 1, i.e. group 3, and day 3, i.e. group 4, n = 3/group). After this, qRT-PCR analysis was done to confirm the results of the microarray study. The rats were again divided into 4 (day 1, i.e. group 1, and day 3, i.e. group 2, n = 5/group), SCI induced (day 1, i.e. group 3, and day 3, i.e. group 4, n = 5/group).
In the 2nd part of the study, the rats were treated with miR-A antagomir. The SCI induced animals were divided into 2 groups, viz. a negative control group (NC) and the miR-A antagomir treated group. The NC group (n = 3) animals were submitted to SCI and were injected with NC antagomir via the intrathecal route 20 nmol/ml). In the 2nd group the SCI subjected rats (n = 3) were given miR-A antagomir (20 nmol/ml) for 3 days. After the protocol of 3 days, the rats from both groups were sacrificed (1 from each group) by overdose of sodium pentobarbital (240 mg/ml); the protocol was in accordance with ethical guidelines. Subsequently, the spinal cord was removed and a long segment measuring 10 mm from the region of injury was harvested. The rats were sacrificed based on the different parameters such as lesion identification by cresyl staining and motor function score at 28 days after SCI. Similarly, expression of target proteins such as FasL, PTEN and PDCD4 was determined on day 1 and day 3 of SCI. The qRT-PCR analysis of miR-A was done on the 1st and the 3rd day after SCI. TUNEL staining and in situ hybridization was done on the 1st and the 3rd day after SCI (Figure 1).
MiRNA microarray analysis
For microarray analysis, the rats after 1 or 3 days of laminectomy were subjected to anesthesia, then a piece of spinal cord about 10 mm long which included the injured portion was harvested and was frozen in liquid nitrogen. The spinal tissues were then submitted to isolation of RNA using TRIzol reagent along with the miRNeasy kit following the supplied instructions. The quantitative and qualitative analysis of RNA and miRNA was performed using a spectrophotometer, whereas the RNA integrity was assessed using gel electrophoresis.
After isolating the RNAs from the spinal tissues, miR labeling was done using the Label IT miRNA Labeling Kit (Mirus Bio, USA) following the manufacturer’s instructions. About 1 µg of each sample was labeled in the 3′ region using the fluorescent labeling reagent from the kit following the supplied instructions. Briefly, 2 µl of aqueous RNA solution was added with 1 µl of alkaline phosphatase and calf intestinal buffer (CIP buffer). The resultant mixture was incubated for 25 min at room temperature followed termination by incubating for 5 min at 95°C. After this about 3 µl of labeling buffer along with 1.5 µl of fluorescent label, 2 µl DMSO and 2 µl of labeling enzyme were added together in the mixture. The final mixture was incubated for 1 h at 16ºC followed by termination by incubating for 20 min at 65ºC.
After the labeling process was completed, the fluorescent labeled samples were subjected to hybridization on an LNA array following the supplied instructions. After the process of hybridization, the slides were collected, rinsed many times using washing buffer and finally were dried in a high speed centrifuge for 5 min at 500 rpm. The slides were scanned and the images were processed for grid alignment followed by extraction of data using GenePix software. For evaluating the normalization factor, the average of replicated miRs was done to calculate the normalization factor.
Administration of miR-19a antagomir and negative control antagomir
The miR-19a antagomir was infused in SCI rats by a catheter using a minipump immediately after inducing spinal cord injury. Briefly, both the minipump and the catheter were implanted by surgery just after the SCI. The miR-19a antagomir and negative control was solubilized in 0.9% saline solution having the concentration 20 nM/ml; the resultant solution was filled in osmotic minipumps followed by continuous infusion in the spinal cord of SCI rats (1 µl/h). After implanting the catheter, surgery was done at the T12 and T13 rat vertebrae. A 32 number catheter was introduced via the dural incision. The catheter was joined to a 2 cm piece of PE10 tubing, which was attached to the osmotic pump. The osmotic pumps in the rats were implanted subcutaneously. Each rat was injected with an intramuscular injection of penicillin (40000 U) during the surgery followed by a once a day dose for the next 5 days. The rats were kept in polypropylene cages and were provided a normal diet along with free access to water.
Locomotor activity by Basso, Beattie and Bresnahan (BBB) locomotor score
The locomotor activity was evaluated by the Basso, Beattie and Bresnahan (BBB) locomotor score. The activity was assessed on the 1st, 3rd, 7th, 14th, 21st, and the 28th day after the SCI induction. The score measured the locomotor activity for 4 min. The score was recorded and compared to the scales provided earlier.
Identification of lesions by cresyl staining method
At the end of the last behavioral test done on the 28th post-surgery day, the rats were euthanized using overdose of sodium pentobarbital (240 mg/ml). The rats were perfused using isotonic solution of sodium chloride (0.9% w/v of NaCl) followed by addition of 500 ml of paraformaldehyde (PFA) (Sigma Aldrich USA) (4%) in 0.1 M PBS (pH 7.4). The injured site and a 1 cm portion of spinal cord was dissected and fixed in fixing media for 24 h at 4ºC. The tissues after fixing were imbedded in paraffin. From the paraffin embedded a section of 10 µM thickness was obtained and mounted on slides. The obtained tissue section was stained with cresyl-violet solution (Sigma Aldrich USA) (0.5%) and viewed under a microscope (Olympus, NY). The lesion areas and the spared tissue areas in the spinal cord tissue sections were identified using Image pro software (Media, USA). The spared tissue area was the part of spinal cord section which showed normal anatomical structure, whereas the area with a low percentage of spared tissue was determined as the injured epicenter.
Quantitative real-time PCR (qRT-PCR)
The injured epicenter of spinal cord was processed and total RNA was isolated using TRIzol reagent. The obtained total RNA from each sample was subjected to reverse transcription to cDNA using a PrimeScript kit (TaKaRa, Japan) followed by qRT-PCR analysis using SYBR primer Ex Taq and miR-19a specific primers. The expression of miRNA levels was normalized to U6 (a small nuclear RNA (snRNA)) expression for each sample; the 2ΔΔCt method was used for gene expression.
Western blot analysis
The animals were subjected to euthanasia by overdose of sodium pentobarbital (240 mg/ml) divided into day 1 and 3 after injury. The spinal cords were harvested and a segment measuring 1 cm with the injured site was used for studying the expression of proteins by western blot. The harvested spinal tissues were homogenized and subjected to lysis, and total protein was determined using protein estimation kit (Sigma Aldrich USA). About 20 µg of protein was submitted to electrophoresis on sodium dodecyl sulfate/polyacrylamide gel (12%) and then transferred to PVDF membranes (Thermo Fisher USA). The PVDF membranes were blocked using 5% nonfat milk for 1 h. The membranes were incubated for 12 h at 4ºC along with rabbit anti-FasL antibody (1 : 1000, Abcam USA), rabbit anti-PTEN antibody (1 : 1000, Abcam USA), rabbit anti-PDCD4 antibody (1 : 1000 Abcam USA) and β-actin (1 : 1000, Abcam, USA). The membranes were then incubated with horseradish peroxidase conjugated IIry antibody (1 : 10000) for 60 min at 37ºC. The proteins were viewed using enhanced chemiluminescence; β-actin was used as loading control.
Immunohistochemical staining
The rats were subjected to euthanasia as described earlier on day 1 and 3 after spinal cord injury (n = 3/group). A segment of spinal cord with the injured site measuring 10 mm was harvested. The isolated spinal tissue was fixed and embedded in paraffin, and sections of 5 µm thickness were obtained. The sections were deparaffinized with xylene and hydrated with alcohol. The unmasking of epitope was microwave assisted in 10 mM citrate buffer at pH 6 at 800W for 3 min followed by cooling for 30 min. The endogenous peroxidase was inactivated by incubating for 10 min in 3% H2O2 at room temperature. The sections were washed at least 2 times with phosphate buffer saline (0.01M) and were blocked using 10% fetal bovine serum for 30 min followed by incubation for 12 h at 4ºC along with Iry antibodies named anti-PTEN (1 : 1000), anti-FasL (1 : 1000) and anti-PDCD4 (1 : 1000). The tissue sections were then incubated with IIry antibodies (IgG antibody). Diaminobenzidine (DAB) staining was carried out to determine the immunoreactivity. The slides were covered with a cover slip and were analyzed under a light microscope.
TUNEL assay
The prepared slides of spinal tissues were used for studying neuronal cell apoptosis by TUNEL assay. The TUNEL staining was done using a TUNEL assay kit (Abcam, USA). The sections were dewaxed by xylene and then rehydrated using alcohol; the sections were placed in water. The processed spinal tissue sections were incubated for 10 min with 20 µg/ml proteinase K working solution at room temperature. The slides were washed 3 times with phosphate buffer saline before incubating with TUNEL mixture for 60 min at room temperature. The sections were washed with phosphate buffer saline and incubated with HRP-streptavidin reagent (1 : 200) in phosphate buffer saline for 30 min at 37ºC. The sections were washed again with phosphate buffer saline 3 times and the sections were incubated with a solution mixture of H2O2 (0.03%) and DAB (0.04%) for 10 min. The sections were again washed with phosphate buffer saline and then counterstained with hematoxylin. The sections were washed 2 times with de-ionized water and mounted on slides with a cover slip. The TUNEL positive cells were quantified from each section twice using a light microscope. Briefly, 2 spinal cord tissue sections were chosen for each animal, 5 areas were randomly selected and the cell counts were done manually; positive cells were calculated.
In situ hybridization
For in situ hybridization, the slides were prepared as described earlier. Briefly, the tissue sections were dewaxed by xylene and rehydrated in alcohol and exposed to an aqueous solution of diethyl pyrocarbonate (DEPC). Inactivation of endogenous peroxidase was done by incubating the sections in H2O2 (3%) for 10 min. The tissue sections were then treated with proteinase K for 25 min and were washed with sodium chloride Tris buffer and fixed using PFA (4%) for 15 min. The slides were again washed with phosphate buffer saline, and the slides were blocked with hybridization buffer (Thermo Fisher USA). The slides were washed after the process of hybridization and incubated with HRP conjugated anti-DIG antibody. The sections were washed with phosphate buffer saline 3 times. DAB was used to visualize peroxidase staining. The sections were washed again with phosphate buffer saline 3 times and stained with hematoxylin. The cells were counted manually in 5 random areas of sections; positive cells were visualized.
Results
Abnormal expression of miRNAs in injured spinal cord
To find the potentially involved miRs in spinal cord injury, we employed microarray analysis to trace the levels of miRs in spinal cord tissues subjected to contusion spinal cord injury. The results suggested that, compared to sham operated rats, about 8 miRs were upregulated whereas 4 were downregulated in rats subjected to spinal cord injury in the day 1 group (Figure 2 A). On comparing sham operated rats in the day 3 group two miRs were overexpressed and four were downregulated in spinal cord injured rats (Figure 2 A). Among the identified miRs, miR-23, miR-24a, miR-126 and miR-19a were abnormally expressed; these miRs were further subjected to qRT-PCR analysis (Figure 2 B). From these miRs, the microarray and RT-PCR analysis suggested that miR-19a was the most deregulated; also miR-19a has been reported to exert protective action in many models of injury [10–13, 15]. Hence, we targeted our study on miR-19a in spinal cord injury for further study.
Figure 2
Cluster analysis (heat map) for alterations in expression of miRNAs after spinal cord injury. A – Cluster analysis shows expression of differentially expressed miRs in sham operated as well as in spinal cord injured rats, 3 days after injury (n = 3 in each group). Cluster analysis showing differential expression of miRs in sham operated and spinal cord injured group of rats on day 1 after injury (n = 3 in each group). The color pattern in the heat map is linear: the green color represents the lowest whereas the red shows the highest; color green to red represents upregulated miRs whereas color red to green indicates down-regulated miRs. B – Results of qRT-PCR analysis confirmed the results of microarray analysis in rats after day 1 and 3 after spinal cord injury. The results were compared to the sham operated group (day 1 and day 3 post injury)
*P < 0.05 and **p < 0.01.
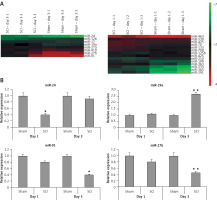
miR-19a antagomir exerts an inhibitory effect on miR-19a
To determine the inhibitory effect of miR-19a antagomir on expression of miR-19a in vivo, we did in situ hybridization using an LNA-containing antisense oligonucleotide probe followed by qRT-PCR analysis. We found that miR-19a was present in the nuclei of many cells compared to the negative control (Figure 3). The miR-19a antagomir decreased the count of miR-19a positive cells in day 1 and 3 groups of rats (Figures 3 A, B, D). The results of qRT-PCR were in parallel to findings of in situ hybridization and suggested that levels of miR-19a decreased significantly in day 1 and day 3 groups in RNAs of rats treated with miR-19a antagomir compared to the negative control (Figure 3 C).
Figure 3
miR-19a antagomir blocks the in vivo expression of miR-19a after spinal cord injury. The results of qRTPCR and situ hybridization for expression of miR-19a after 1 and 3 days after spinal cord injury. miR-19a was localized in the nucleus of cells after spinal cord injury. The miR-19a antagomir decreased the number of miR-19a positive cells significantly as compared to the negative control group after 1 day (A, D) and 3 days after spinal cord injury. The findings of in situ hybridization and qRT-PCR were parallel to each other and showed that expression of miR-19a decreased significantly on day 1 and day 3 in rats subjected to spinal cord injury and treated with miR-19a antagomir compared to the negative control group (B, D)
*P < 0.05, **p < 0.01.
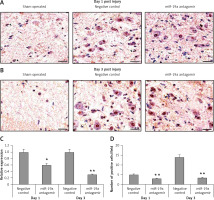
Inhibition of miR-19a aggravated the functional deficit mediated by spinal cord injury
The rats after contusion spinal cord injury were paralyzed from both the hind limbs. The rats showed recovery of spontaneous function after spinal cord injury in day 1 and day 3 groups (Figure 4). We observed that the hind limb locomotor improved during the experimental period as seen by the increase in BBB score in both the groups. The treatment of miR-19a antagomir attenuated the injury after the 14th day along with the negative control group. After 4 weeks of spinal cord injury, the BBB scores suggested that miR-19a antagomir exacerbated the movement of animals subjected to spinal cord injury.
Figure 4
Blockade of miR-19a aggravated the functional deficit after spinal cord injury in rats. The hindlimb was assessed using Basso, Beattie, and Bresnahan (BBB) Score for recovery from the 1st day to the 28th day after spinal cord injury. The dysfunctioning of hindlimb was aggravated when treated with miR-19a antagomir compared to the negative control
*P < 0.05; **p < 0.01.
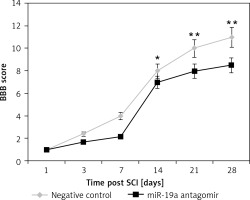
Inhibition of miR-19a aggravated tissue damage in the injured spinal cord
To evaluate the lesion size, cresyl violet staining was done for spared spinal tissue followed by behavioral studies after 28 days of spinal injury (Figure 5). The sections from the thoracic region of the spinal cord were evaluated; the injured area from the total area was compared for each tissue section (Figure 5 A). The study of sections suggested that the miR-19a antagomir treated rats showed a large lesion not only in the injured area, but also in the area stretching from the epicenter in both the caudal and rostral direction. The miR-19a antagomir treated rats when compared to negative control rats showed a significantly small spared tissue area around the lesion epicenter in the caudal and rostral direction (Figures 5 B, C).
Figure 5
Blockade of miR-19a exacerbates the tissue damage of spinal tissues in spinal cord injured rats. A – The spinal cord sections were stained with cresyl violet and were evaluated for size of lesion and spared tissue after 28 days after spinal injury. The sections show spared tissue and lesion size from the injured site and the area 1600 μm rostral and caudal to the injured site after 28 days of injury. B – The results of quantitative analysis of spared tissue around the injured site and 1600 μm rostral and caudal to the epicenter after 28 days of injury. C – Quantitative analysis of lesion size within the injured area and 1600 μm rostral and caudal to the epicenter after 28 days of injury
*P < 0.05; **p < 0.01.
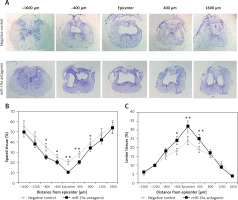
Downregulated expression of miR-19a increased apoptotic cells after spinal cord injury
TUNEL staining assay was done to study the effect of miR-19a on apoptotic cells after spinal cord injury. Quantification was done after day 1 and day 3 after injury. We noted that most of the TUNEL positively stained cells were present in the gray matter and a few of the TUNEL positive cells were observed adjacent to white matter. The number of positive stained cells was high in the spinal cord tissue section observed in day 3 post-injury rats and the negative control group. When compared to negative control group rats, the miR-19a antagomir treated rats at day 3 after injury showed a significant increase in the number of TUNEL positive cells compared to the negative control (Figure 6).
Figure 6
Decreased expression of miR-19a enhances the apoptotic cell death after spinal cord injury. A, B – Microscopic images of spinal cord tissue section subjected to TUNEL assay to analyze apoptosis of neurons after spinal cord injury on post-injury day 1 and day 3 in miR-19a antagomir and negative control groups. It was observed that miR-19a antagomir treated spinal cord injured rats after 3 days after injury showed a higher number of TUNEL positive cells compared to the negative control
*P < 0.05.
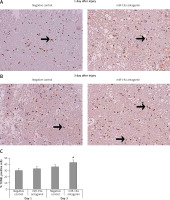
Blockade of miR-19a did not alter expression of PDCD4 but increased levels of FasL and PTEN in vivo
PDCD4, FasL and PTEN were the established targets of miR-19a; hence we targeted these genes and postulated that they may be regulated by miR-19a in vivo. Expression of PTEN, FasL and PDCD4 was evaluated by western blot analysis. It was observed that treatment of miR-19a antagomir compared to the negative control resulted in a significant increase in expression of FasL at the 3rd day after injury, but the levels of FasL were the same on the 1st day compared to the negative control (Figure 7). Similarly, treatment with miR-19a antagomir elevated the levels of PTEN compared to the negative control group on the 3rd day, but no significant changes were seen on the 1st day in both groups (Figures 8 C, D). The results of immunohistochemical staining suggested that PTEN was localized in cytoplasm and upregulated after treatment with miR-19a antagomir on the 3rd day, the expression was parallel in both the groups on the 1st day, and the results were parallel to the findings of western blot. The results also showed that treatment with miR-19a antagomir had no any affect on the expression of PDCD4 after spinal cord injury (Figures 9 C, D). The results of immunohistochemical analysis suggested that PDCD4 was localized in the cytoplasm region, whereas the expression of PDCD4 was identical at the 1st and the 3rd day; the findings were parallel to the outcomes of western blot analysis.
Figure 7
Blockade of miR-19a increased the expression levels of FasL gene. A – Western blot analysis for expression of FasL after spinal cord injury, in rats treated with miR-19a antagomir and negative control group. B – Quantitative analysis for optical density of expression was measured, the expression after treatment with miR-19a antagomir significantly increased FasL after day 3; the levels of FasL were similar in both groups after day 1
**P < 001 compared to negative control.
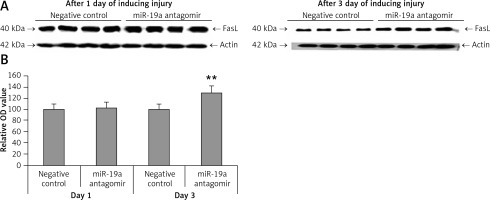
Figure 8
Blockade of miR-19a enhances the expression of PTEN. A, B – The levels of PTEN after spinal cord injury were identified by immunohistochemical staining in the spinal cord tissue from miR-19a antagomir and negative control. It was observed that PTEN was localized mainly in the cytoplasm of neurons. (A) The staining was similar in both groups after day 1, whereas (B) the treatment of miR-19a antagomir caused deep staining against the negative control. C – Western blot analysis was done for expression of protein levels of PTEN after spinal cord injury after day 1 and day 3. D – The expression was determined by densitometry analysis. The treatment with miR-19a antagomir enhanced the expression of PTEN compared to the negative control group after day 3; no changes were seen after day 1
*P < 0.05 compared to negative control.
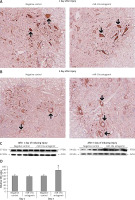
Figure 9
Blockade of miR-19a exerts no effect on the expression of PDCD4. A, B – Immunohistochemical analysis for expression PDCD4 in spinal cord injured in miR-19a antagomir and negative control. PDCD4 was localized in cytoplasm of cells; the staining was similar in both the groups (post-injury day 1 and day 3). C, D – Western blot analysis was done to evaluate the levels of protein PDCD4; densitometry analysis was carried for each band. It was noted that treatment of miR-19a antagomir had no effect on levels of PDCD4 after spinal cord injury
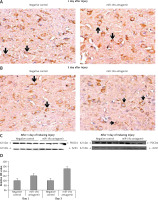
Discussion
Spinal cord injury results in major biochemical and molecular changes which include alterations in expression of mRNA such as gadd45a, c-myc, cyclin D1 and cdk4, pcna, cyclin G, Rb, and E2F5. The genes associated inflammation, oxidative stress, DNA damage, and cell cycle are also found to be altered [16, 17]. Several studies have been done to increase our understanding of expression of mRNA and involved proteins following spinal cord injury and how they correlate with the pathophysiology of spinal cord injury. Recently, researchers have demonstrated that individual miRs can modulate numbers of genes simultaneously by targeting mRNAs and inhibit translation [18]. Hence, alterations in expression of miRs may affect the pathophysiology of spinal cord injury. By performing miRNA microarray analysis, it was found that miR-23, miR-24a, miR-126 and miR-19a were aberrantly expressed in spinal cord injured rats; the results were further confirmed by qRT-PCR analysis. It is to be noted that a number of studies have emerged which have demonstrated aberrant expression of miR-19a in animal models of injury. In a study, miR-19a was found to be over-expressed in ischemic stroke [9]. In a study by Ge et al., miR-19a attenuated cerebral ischemic injury by targeting ADIPOR2, identifying it as a novel target for treating ischemic brain injury [10]. Findings also have confirmed that expression of a number of miRs was altered in the hippocampus region of the brain after traumatic brain injury [22]. Similar to this, our results demonstrated that miR-19a was one of the aberrantly expressed miRNAs after spinal cord injury. miR-19a has been reported to show a potential anti-apoptotic effect in cardiomyocytes and has been found to protect cardiomyocytes from hypoxia-induced apoptosis through the PTEN/p-Akt pathway [23]. In the present study, we found that expression of miR-19a was significantly upregulated in rats after 3 days of spinal injury, inhibition of miR-19a aggravated the tissue damage and functional deficit and enhanced the apoptotic cell death mediated by spinal cord injury. These findings indicate that miR-19a exerts a protective effect in spinal cord injury via inhibiting the cell death of neurons.
miR-19a demonstrated its anti-apoptotic effect by targeting the pro-apoptotic genes. Fas ligand (FasL) is a type-2 category transmembrane protein which belongs to the TNF-α. TNF-α is identified to be one of the potential targets of miR-19a. miR-19a, which belongs to the miR17-92 cluster, has been shown to decrease the expression of FasL in ischemic neural progenitor cells [24]. Overexpression of miR-19a in an animal model inhibited the ischemia-mediated upregulation of FasL, and attenuated apoptosis and heart failure [20]. It has already been evidenced that in many neurological disorders, expression of FasL is upregulated [25–27]. In addition, FasL has a major role in apoptotic cell death after spinal cord injury [25, 27]. Our results suggested that inhibition of expression of miR-19a in an animal model upregulated the expression of FasL. Also we found that miR-19a played an anti-apoptotic role via targeting FasL after spinal cord injury.
PTEN is identified to be one of the potential targets of miR-19a [15]. miR-19a-induced regulation of PTEN has been demonstrated in malignancies such as hepatic cancer, ovarian cancer and myeloma [28–30]. Studies also have established that inhibition of PTEN led to development of uninjured axons in the corticospinal tract, and also led to successful regeneration of injured axons in spinal cord lesion after spinal cord injury [31]; this also increased the survival of neurons [32, 33]. Our findings demonstrated that inhibiting the expression of miR-19a elevated the expression of PTEN and increased the size of the lesion and decreased the spared tissue. The findings suggested that miR-19a may promote generation of neurons and increase the survival of neurons via targeting PTEN after spinal cord injury.
PDCD4 is another important target of miR-19a. PDCD4 is identified to be a tumor suppressor and is found to be downregulated in number of malignancies and is overexpressed during the process of apoptosis [34–36]. PDCD4 is also upregulated in a number of diseases and cell types. Studies have demonstrated that expression of PDCD4 is elevated after inhibition of miR-19a, and miR-19a has a direct binding site on the 3′-UTR region of PDCD4 [33, 37, 38]. Negative regulation of PDCD4 via miR-19a resulted in a protective effect against ischemia-mediated reperfusion injury in heart [23]. In our study, we found that levels of PDCD4 were not altered after inhibiting the expression of miR-19a. The results suggest that though miRs may have different and numerous targets, their modulation may depend or vary based upon the tissue or cell specificity.
In conclusion, the study concludes that spinal cord injury may show a varied pattern of expression of miRs. Among them we found that miR-19a was aberrantly expressed in rats submitted to contusion spinal cord injury. Inhibition of miR-19a in rats aggravated the functional deficit, led to tissue damage and increased apoptotic cell death in animal models of spinal cord injury. We also confirmed that miR-19a possibly exerts its anti-apoptotic effect via targeting the two pro-apoptotic genes PTEN and FasL. These findings suggested that miR-19a may play a crucial role in pathophysiology of spinal cord injury. As miRs are highly unpredictable regulators having an impact on a number of target genes, the exact role of miR-19a in spinal cord injury involving other models needs to be explored.