Introduction
In various acute and chronic renal inflammatory conditions, proximal renal tubular epithelial cells (PRTECs), as intrinsic renal cells, are not only the victim of but also actively participate in the development of glomerulosclerosis and fibrosis by secreting various inflammatory chemokines and extracellular matrix [1]. Studies in the past three years have found that the renal inflammatory microenvironment and fibrosis are closely related to the occurrence of renal cancer. Renal cancer is a malignant tumor originating from the renal parenchyma urotubular epithelial system. Inflammatory changes in PRTECs and the release of inflammatory factors may play a role in promoting tumors [2–6].
Curcumin is a class of plant polyphenols extracted from ginger plants, such as turmeric and Curcuma zedoaria rhizomes, with various pharmacological activities, such as anti-inflammatory, antioxidant, hypolipidemic, antitumor, and immunomodulatory [7]. Curcumin plays a role in multiple cancers [8]. Curcumin can affect cellular redox balance, resulting in increased production of reactive oxygen species (ROS), and excess ROS can affect cell death patterns [9].
The ADAMTS family consists of 19 secreted multidomain zinc finger metalloproteinases. Dysregulated ADAMTS18 expression is associated with many diseases, such as inflammation, cancer, arthritis, and atherosclerosis [10]. Our research group has studied ADAMTS18 in depth in the past 10 years, especially in the field of renal cancer. We found that ADAMTS18 can affect renal cells through abnormal DNA methylation [11, 12], and curcumin can affect this process through the regulation of ADAMTS18 [13–15].
This study investigated the effect of curcumin on cell viability after LPS-induced PRTEC inflammatory lesions. We explored whether curcumin affects LPS-induced PRTEC activity by regulating ADAMTS18 methylation and AKT/Notch pathways.
Material and methods
Experimental materials
The human renal proximal tubular epithelial cell line (HK-2) was purchased from the cell bank of Peking Union Medical College Hospital. LPS and curcumin were purchased from Sigma Corporation. The cells were cultured in RPMI 1640 supplemented with 20% bovine fetal serum, 5 μg/ml transferrin, 5 ng/ml sodium selenite, 5 μg/ml insulin, streptomycin (0.1 mg/ml), and penicillin (100 U/ml). Cells were cultured and passaged in a 37°C, 5% CO2 incubator. HK-2 cells were cultured in serum-free medium containing 0.1% bovine serum albumin for 24 h and then treated with LPS (Sigma, USA) [16]. The protein detection kits used in this experiment were from Beijing Sangon Biotech Co., Ltd.
RT-qPCR
RNA was extracted from the target tissues and cells, and cDNA was synthesized by reverse transcription. After PCR, the amplicons were separated by agarose gel electrophoresis, and the ImageJ gel image system was used. The expression level of ADAMTS18 mRNA was analyzed using 3-phosphoglyceraldehyde dehydrogenase (GAPDH) as the reference gene. The qPCR primers were ADAMTS18 (F 5′-3′ GACACAGACAGACGCACAGA, R 5′-3′ TCCTGCCGTTGTGCAAAATG), GAPDH (F 5′-3′ GGAGCGAGATCCCTCCAAAAT, R 5′-3′ GGCTGTTGTCATACTTCTCATGG).
Western blotting
Cell lysate proteins were separated by 12% sodium dodecyl sulfate–polyacrylamide gel electrophoresis, transferred to nitrocellulose membranes, and treated with antibodies, then incubated at 4°C for 12 h. The membrane was washed five times with TBST buffer solution and incubated with goat anti-rabbit or anti-mouse IgG for 2 h at room temperature. Excess antibody was removed by washing with TBST, after which specific binding was detected with a chemiluminescence detection system.
Methylation-specific PCR (MSP)
Sulfite-modified DNA was used as a template to design methylation- and nonmethylation-specific primers with the help of Primer 5.0 software. Hot-start PCR amplification was performed in cell lines with deionized water as a blank control. After amplification, 10 μl of MSP amplification products were separated by 2% agarose gel electrophoresis and stained in ethidium bromide solution. The results were observed and the images were captured under ultraviolet light.
MTT method
HK-2 cells were seeded in 96-well plates (1 × 104 cells/well) and treated with curcumin for 12 h. Then, 10 μl (0.5 mg/ml) of MTT was added to each well, followed by incubation at 37°C and 5% CO2 for 2 h. The supernatant was removed, 100 μl of dimethyl sulfoxide was added, and the absorbance was measured at 490 nm using a UV MAX dynamic microplate reader.
Flow cytometry
HK-2 cells were inoculated in a six-well plate (2 × 105 cells/well) and treated with curcumin. Then the cells were processed with the Annexin V FITC Apoptosis Detection Kit according to the kit instructions and analyzed using fluorescence microscopy and flow cytometry.
Transfection of siADAMTS18
HK-2 cells were transfected with ADAMTS18 siRNA or siNC as a negative control (Santa Cruz, CA, USA) at a final concentration of 40 nM using Lipofectamine 2000 (Invitrogen) according to the manufacturer’s instructions. The transfection efficiency was verified by real-time RT-PCR.
Enzyme-linked immunosorbent assay (ELISA)
A one-step ELISA kit (Thermo Fisher Scientific) was used according to the manual. It included seven steps: activation of sample plate, adding samples, incubation, washing, adding TMB substrate, adding stop solution, and calculating the results.
Statistical analysis
Experiments were done in at least three independent replicates, and the data are presented as the mean ± standard error of the mean (SEM). All of the tested variables were normally distributed. Two-way analysis of variance was run to analyze the differences between the groups in the experiments, after which Tukey’s test was used to determine the statistical significance between two groups (α = 0.05). Statistical analysis was performed with SPSS software. P < 0.05 was considered statistically significant.
Results
Effect of curcumin on cell viability
MTT assay showed that LPS stimulation of HK-2 cells significantly reduced cell viability (p < 0.05). After curcumin incubation, the activity was significantly increased (p < 0.05) (Figure 1 A). Flow cytometry showed that the apoptosis rate was significantly increased with LPS induction (p < 0.05). The apoptosis rate was significantly decreased after the addition of curcumin (p < 0.01) (Figures 1 B–E). These results suggested that curcumin had a certain protective effect against the LPS-induced apoptosis of HK-2 cells (Figure 1 F).
Figure 1
The effect of curcumin on cell viability. A – MTT assay was used to determine the effects of LPS and curcumin on HK-2 cells; B – Flow cytometry analysis of cell apoptosis in curcumin + DMSO group; C – Flow cytometry analysis of cell apoptosis in curcumin + LPS group; D – Flow cytometry analysis of cell apoptosis in DMSO group; E – Flow cytometry analysis of cell apoptosis in LPS group; F – Comparison of apoptosis rates using bar charts
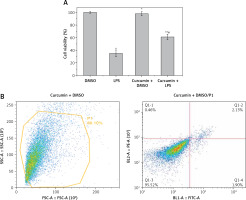
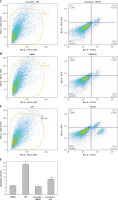
Effect of curcumin on ADAMTS18
The expression of ADAMTS18 was detected by RT-qPCR (Figure 2 A) and WB (Figure 2 B). In the MSP assay of the level of ADAMTS18 methylation, curcumin had a demethylation effect on ADAMTS18 (Figure 2 C).
Effect of ADAMTS18 on cell viability
HK-2 cells were transfected with siADAMTS18 or the blank control siNC. RT-qPCR showed that the transfection was successful, and the expression of ADAMTS18 was significantly downregulated by siADAMTS18 (p < 0.01) (Figure 3 A). The cells were divided into siNC, siNC + curcumin, siADAMTS18, and siADAMTS18 + curcumin groups, and each group was treated with LPS. Curcumin significantly increased the ADAMTS18 activity. Compared with the siNC group, the ability of curcumin to improve the activity decreased in the siADAMTS18 group (p < 0.05) (Figure 3 B). Flow cytometry showed that LPS promoted apoptosis, while curcumin reduced the apoptosis rate (p < 0.01). There was no difference in the percentage of apoptosis induced by LPS between siRNA groups (p > 0.05), but the protective effect of curcumin was reduced in the siADAMTS18 group (p < 0.05) (Figures 3 C–F). These findings suggested that curcumin increased the activity of HK-2 cells and reduced LPS-induced apoptosis, which may be related to the increase in ADAMTS18 expression allowed by demethylation of ADAMTS18 (Figure 3 G).
Figure 3
Effect of ADAMTS18 on cell viability. A – siADAMTS18 was successfully constructed; B – MTT assay to determine the effect of LPS and curcumin on HK-2 cells; C – Flow cytometry analysis of cell apoptosis in LPS + siADAMTS18 group; D – Flow cytometry analysis of cell apoptosis in LPS + siADAMTS18 + curcumin group; E – Flow cytometry analysis of cell apoptosis in LPS + siNC group; F – Flow cytometry analysis of cell apoptosis in LPS + siNC +curcumin group; G – Comparison of apoptosis rates using bar charts
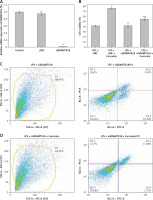
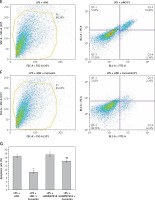
Curcumin inhibits the expression of inflammatory factors
ELISA was used to analyze the levels of IL-1β, IL-6, IL-8, and TNF-α in the cell culture medium of each group. The results are shown in Figures 4 A–D. The changes in IL-1β, IL-6, IL-8, and TNF-α were consistent with each other, curcumin significantly reducing the expression of inflammatory factors. With siADAMTS18, the expression of inflammatory factors was still low after curcumin treatment, but the reduction was smaller than that in the siNC group (p < 0.05), indicating that the ability of curcumin to reduce cellular inflammatory factors was inhibited by ADAMTS18 knockdown, which again suggests that curcumin may protect cells through ADAMTS18.
Effect of curcumin and ADAMTS18 on AKT/Notch pathways
To find out how curcumin inhibits the death of HK-2 cells, the expression of AKT, Notch-1 and Notch-2 was analyzed (Figure 5 A). The results indicated that curcumin treatment of HK-2 cells reduced the levels of AKT, Notch-1 and Notch-2. With siADAMTS18, the inhibitory effect of curcumin on AKT, Notch-1 and Notch-2 was reduced (p < 0.01) (Figures 5 B–D). In conclusion, siADAMTS18 attenuated the effect of curcumin interference.
Discussion
When inflammation occurs in PRTECs, they promote the occurrence and progression of various kidney diseases by secreting inflammatory factors and extracellular matrix, which are closely related to fibrosis. The occurrence and progression of renal cancer are also closely related to the inflammatory microenvironment and fibrosis. Our previous studies in renal cancer found that ADAMTS18 expression is closely related to the occurrence, development, invasion, and metastasis of renal cancer [11, 12], and it is hypothesized that ADAMTS18 is also related to the expression of inflammatory factors in renal cells, thereby regulating the death of normal renal cells. The purpose of this study was to use LPS to induce a renal inflammatory cell model and then knock down ADAMTS18 to explore the mechanisms underlying its effect on inflammatory factors and renal cell activity. LPS has been intensively used as an effective tool to induce inflammation and thus explore a novel biomarker of the inflammatory response [17, 18]. Related studies have reported that PRTECs, as early and important target cells of LPS, can be used to study various renal inflammatory responses [19].
In the treatment of renal inflammatory diseases, traditional herbal medicines have been studied in depth due to their large quantity, good safety, and low price. Curcumin, a class of polyphenols extracted from the rhizomes of turmeric plants, has been identified to exert anticancer effects on liver carcinoma and inhibit inflammatory diseases in liver diseases [20, 21]. Mollazadeh et al. proposed that in vitro and in a pre-clinical model curcumin is able to modulate the disease pathophysiology of conditions such as pain and neurodegenerative diseases, bowel inflammation, and allergy, but also of infections and cancer through its effect on IL-10 secretion [22]. Shakeri et al. also reported that curcumin as a hydrophobic polyphenol compound extracted from the known spice turmeric has different pharmacological effects in both in vitro and in vivo models, which is capable of triggering autophagy in several cancer cells [23]. Ahmadabady et al. found that curcumin can improve LPS-induced oxidative stress and inflammation in rats and protect cardiovascular function [24]. Li et al. proposed that curcumin can reduce LPS-induced accumulation of ROS in bovine mammary epithelial cells, reduce the expression of inflammatory factors such as TNF-α, and reduce the apoptosis rate [25].
Our research group has been working on the inhibitory effect of curcumin on renal cancer for the past 2 years and found that curcumin can inhibit the occurrence and progression of renal cancer by regulating ADAMTS18 and can inhibit the release of inflammatory factors in the process. We hypothesize that curcumin protects normal renal cell activity by regulating ADAMTS18 to suppress the inflammatory response.
Working from the above hypothesis, this study demonstrated that curcumin can inhibit LPS-induced inflammatory lesions in HK-2 cells, promote cell proliferation, and reduce their apoptosis rate. Curcumin exerted a protective effect on HK-2 cells by down-regulating ADAMTS18 methylation. Upregulation of ADAMTS18 restored normal renal cell activity. This study found that the ability of curcumin to improve cell activity was reduced after ADAMTS18 knockdown, indicating that curcumin-mediated reduction of LPS-induced death may require its regulation of ADAMTS18 expression. ELISA showed that IL-1β, IL-6, IL-8, and TNF-α were significantly reduced after curcumin treatment, while the ability of curcumin to downregulate inflammatory factors was significantly reduced after ADAMTS18 knockdown, which confirms that curcumin reduces inflammatory lesions through ADAMTS18. Additionally, WB showed that curcumin induced down-regulation of AKT, Notch-1 and Notch-2 levels, which suggests that curcumin might inhibit LPS-induced PRTECs death by regulating AKT/Notch pathways. This is consistent with previous research results: Ying et al. discovered that the AKT signal pathway is directly related to cell apoptosis [26]. Hao et al. proposed that the phosphoinositide 3-kinase (PI3K)/AKT signaling pathway is implicated in multiple cellular processes, such as survival, proliferation, differentiation, and apoptosis, and the PI3K/Akt signaling pathway is linked to major degenerative diseases [27].
In conclusion, curcumin inhibits LPS-induced PRTEC death by regulating ADAMTS18 methylation and AKT/Notch pathways.